Introduction
Atrial fibrillation (AF) ablation has repeatedly proven its efficacy in the treatment of drug resistant forms of the arrhythmia [1–3]. Although, pulmonary vein isolation (PVI) is the cornerstone in today’s everyday practice, a considerable proportion of patients will recur with atrial arrhythmias following this procedure. Various strategies such as ablation of complex fractionated atrial electrograms (CFAE), ganglionated plexi, rotors, AF “nests”, or creation of lines in the left atrium have been proposed as adjunctive techniques to PVI, especially in patients affected by more advanced forms of AF [4–9]. Nevertheless, the results are controversial, and the optimal ablation strategy that might lead to significantly better results still needs to be determined.
Cardiac nervous system and atrial fibrillation
Anatomically, the cardiac nervous system can be subdivided into the extrinsic cardiac nervous system (ECNS), which contains fibers that mediate connections between the heart to the brain and the spinal cord, and the intrinsic cardiac nervous system (ICNS), composed primarily by autonomic nervous fibers, which is an interconnected neural system consisting of epicardial ganglionated plexi (GPs) (Figure 1) and an extensive network of atrial intramural microganglia [10, 11].
Figure 1
Scheme of cardiac autonomic nervous system. Schematic of the cardiac autonomic nervous system showing the difference between the parasympathetic and the sympathetic innervation, regarding the distance of the neural body from the heart
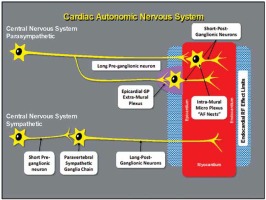
GPs are not merely “relay stations” for ANS projections to the heart; they contain part of the sympathetic efferent axons, almost all parasympathetic efferences, sensory afferences, and local circuit neurons, that contribute to the coordination of regional cardiac electrical and mechanical indices on a beat-to-beat basis [12].
Vagal activation might play a pivotal role in the pathogenesis of AF [13]. In 1978, a publication first proposed that AF may have a nervous system trigger [14]. Subsequently, 2 subtypes of AF were described. First subtype characterized by bradycardia and therefore defined as vagotonic AF, and second subtype induced by an increased sympathetic tone. The first subtype was often documented in young, healthy individuals, with no apparent structural heart disease; the second subtype was usually depicted in older individuals, with multiple co-morbidities and structural heart disease [15].
Various studies have shown the importance of the extrinsic and intrinsic cardiac autonomic nervous system (ICANS) innervation in the genesis and maintenance of atrial fibrillation [16–20]. With this rationale in mind, ablation of ganglionated plexi in addition to PVI has been recently proposed in order to improve clinical outcomes.
The parasympathetic postganglionic neuronal cells are found both in the paracardiac ganglia and in the cardiac wall [21, 22]. As a consequence, axons are short in length and the ablation of these structures makes parasympathetic reinnervation less likely, as the neuron body is eliminated [7] (Figures 2 and 3). This action might enable permanent ablation or attenuation of these ganglia from the endocardial surface.
Figure 2
Schematic sites of the epicardial ganglionated plexis. Schematic view of the epicardial ganglionated plexis corresponding to ganglion 1, between the superior vena cava and the aortic root just above the right upper pulmonary vein; ganglion 2, between the right upper pulmonary vein and the right atrium; ganglion 3, between the inferior vena cava and the right/ left atrium; ganglion 4, between left pulmonary vein and left pulmonary artery. Attention must be paid to the anatomical similarity between the Ganglions and the usual ablation sights of wide circumferential ablation for pulmonary isolation. 1, 2, 3, and 4-ganglionated plexis
Ao – aorta, IVC – inferior vena cava, LA – left atrium, LV – lefty ventricular, PA – pulmonary artery, RA – right atrium, RV – right ventricular, SVC – superior vena cava, dotted line – usual ablation sights.
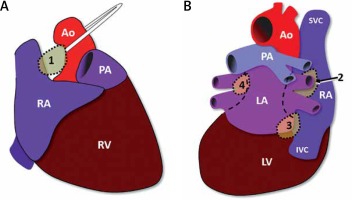
Parasympathetic denervation
Parasympathetic denervation (PD) is being increasingly performed as an adjunctive ablation technique to PVI in the setting of percutaneous treatment of AF in order to potentially improve outcome [5–7, 23–25], but the success of this strategy depends on an accurate definition of the target regions and consequently, a reliable proof of denervation.
In 2005, a new technique was proposed for parasympathetic denervation (cardioneuroablation) [26] by using conventional endocardial RF ablation (Figure 3), and in 2015, a specific technique was published to confirm the vagal tone elimination just after the ablation [27].
Therefore, a reproducible technique affording a reliable evaluation of cardio-neuromodulation (cardioneuroablation) during and at the end of the procedure is mandatory [27]. Techniques aiming at specific pre-ablation mapping may be considered such as the spectral-guided ablation by fast Fourier transform (FFT) analysis [7], the simplified spectral analysis by specific filtering the RF-catheter signal in 3 channels of the traditional EP recording system [7], the high-frequency endocardial stimulation (HFS) [28, 29], the anatomic GP ablation [30, 31], and the atropine response abolition [32] (Table I).
Table I
Resume of parasympathetic modulation studies
First author, year [references] | Number of participants and characteristics | Intervention | Outcome | Study design | Mean follow-up duration [months] |
---|---|---|---|---|---|
Pachon, 2004 [7] | 34 paroxysmal or persistent AF | RF ablation targeting “AF nests” with FFT | 94% of the paroxysmal AF free of recurrence | Prospective | 9.9 ±5.0 |
Scannavaca, 2006 [28] | 10 paroxysmal AF | RF ablation with HFS | 5 of 10 remain asymptomatic | Prospective | 8.3 ±2.8 |
Katritsis, 2008 [30] | 19 paroxysmal AF | RF anatomically guided | Arrhythmia recurred in 14 (74%) patients with GP ablation | Prospective | 12.0 |
Pokushalov, 2009 [34] | 40 HFS-guided and 40 anatomical guided | LA selective ablation of GP identified by HFS or LA extensive regional ablation targeting the anatomic areas of GP | 42.5% of patients with selective GP and 77.5% of patients with anatomic ablation were free of symptomatic paroxysmal AF | Randomized | 13.1 ±1.9 |
Calò, 2012 [29] | 34 paroxysmal AF | RA RF ablation with elimination of vagal reflex evoked by HFS or an extensive approach at anatomic sites of GP | AF recurred in 5 of 17 patients with anatomic ablation and in 13 of 17 patients with a selective approach | Randomized | 19.7 ±5.2 |
Pachon, 2015 [27] | 47 denervation group and 17 control group | RF ablation targeting vagal tone reduction or conventional ablation | After ablation, the cardioinhibition was reproduced only in CG as in DG it was entirely eliminated | Prospective | 8.8 ±5.0 |
HFS directly stimulates the neural fibers in the atrial wall [33]. Although reliable during AF, this technique might be more challenging in sinus rhythm. The difficulty of this method lies in the fact that it may be only performed in restricted areas and may cause undesired arrhythmias in case of dislodgement.
An anatomical approach for regional ablation of GPs in the right (RA) [28] and left atria (LA) [34, 35] has proven superior to selective GP ablation, controlled by HFS in maintaining patients free from AF recurrence.
Furthermore, atropine results in important autonomic changes might impede any complementary ablation in the same session. Indeed, regarding the increase of the sympathetic tone following the vagal ablation, the response to atropine has low value, as the heart rate was increased by the ablation.
The spectral analysis using the fast Fourier transform (FFT) in sinus rhythm was used for the first time to localize the insertion of nervous fibers without the need of a vagal response. The latter described 2 different types of atrial myocardium. On the one hand, the compact myocardium, with isotropic conduction, well-connected cells and a smooth spectrum, and on the other hand, the fibrillar type (AF “nests”), with a segmented spectrum and anisotropic conduction due to the afference of the nervous fibers into the myocardium. The authors introduced a new approach for the ablation of AF consisting in targeting AF nests with RF in sinus rhythm, guided by the FFT, which was hypothesized as being the real AF substrate. The mean follow-up was 9.9 ±5.0 months. After a blanking period of 3 months, 32 patients did not present recurrence of AF (94.1%) [7].
Until 2015, a reliable method to confirm the efficacy of vagal denervation following the procedure was lacking. Pachon et al. [27] proposed a simplified method to evaluate the effects of ablation on the vagal innervation by using a customized neurostimulator. In brief, direct current stimulation, with square wave pulses of 50 μs in duration at a frequency of 30 Hz and amplitude (adapted according to patient characteristics, 0.5 to 1 V/kg, limited to 70 V) between 10 to 70 V was delivered through a conventional catheter. The latter was positioned into the internal right jugular vein at the level of the upper wisdom tooth (Figure 4). This resulted in the stimulation of the vagus nerve by an endovascular electrical field. Short stimulations and minor modifications were performed to look for the optimal position corresponding to the highest response of sudden cardioinhibition (sinus arrest or bradycardia and/or atrioventricular (AV) block) induction, while preventing lesions to the surrounding tissues. Shortly after, the same protocol was performed in the left internal jugular vein. The locations corresponding to the best responses were memorized in the antero-posterior fluoroscopic projection. After having identified the ideal stimulating position, 8 to 12 s stimulation were delivered on each side. After the ablation, VS was once more performed.
Figure 4
Vagal stimulation method. Antero-posterior fluoroscopic projection of the catheter into the right jugular vein to acquire relevant closeness to the jugular foramen for vagal stimulation
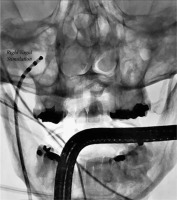
The efficacy of this simplified method was described in a recent publication [27]. The authors compared a “denervation group” (DG), that underwent ablation targeting vagal tone reduction (vagal denervation for treating AF clinically related to vagal tone or severe cardioinhibitory syncope), and a “control group” (CG) submitted to ablations of conventional arrhythmias without aiming at vagal tone modification (Figure 5).
Figure 5
Example of a vagal stimulation and cardiac parasympathetic modulation show the initial vagal response (cardioinhibition of 14 s) after 5 s of an extracardiac vagal stimulation before ablation (A). At the end of the procedure, with the same 5 s of an extracardiac vagal stimulation, the vagal response was practically dismissed (RR interval changed from 834 ms to 868 ms) (B)
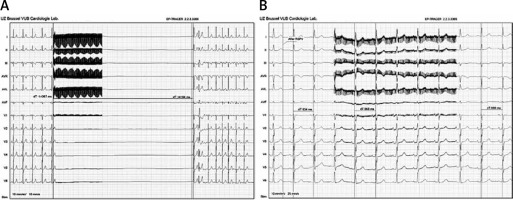
The endpoint of the procedure was characterized by the absence of any sort of vagal response in the DG. Notable cardioinhibition was obtained pre-ablation in all cases (pause of 11.5 ±1.9 s in DG vs. 11.4 ±2.1 s in CG). After ablation, cardioinhibition was reproducible only in the CG (pause of 11.2 ±2.2 s), and in all patients of the DG group in whom the ablation targeted the elimination of the AF nests, the response was completely abolished. There was no vascular injury, case of symptoms, or signs related to neurostimulation in a median (follow-up: 8.8 ±5 months) [27].
Conclusions
Although very promising, these results should not be generalized, and larger randomized trials are needed to confirm the abovementioned findings. However, these techniques that promote extensive vagal denervation through endocardial RF ablation might be of great value in the treatment of AF, especially in cases presenting a clear relation with the vagal tone.