Introduction
B cells are white blood cells (WBCs) in vertebrates. B cells mature in the bone marrow [1] and are a critical element of the acquired immune system. B-cell maturation in the bone marrow is a dynamic and compound procedure involving a steady balance between cell proliferation and apoptotic selection. Due to this balance in the generation of functioning B cells that are liable for eliciting humoral immunity [2–5]. B cells are well recognised to organise immunological responses by antibody production, which represents the humoral arm of the acquired immune system. B cells are the first facilitators of antigen-specific immunological responses by producing antibodies and differentiating into memory cells that give long-standing immunity [6]. B cells positively modulate immunological responses and inflammation by antibody production and antigen presentation for optimal CD4+ T-cell activation [7, 8]. The antibody production enables optimal CD41 T-cell activation as B cells act as antigen-presenting cells and exert different regulatory roles in immunological responses. Nevertheless, specific B cells also negatively modulate the immunological responses through the production of modulatory cytokines and primarily interact with pathogenic T cells via cell-to-cell communication. These categories of B cells are termed “Bregs” [5]. Over the past decade, the functions of Bregs in repressing pathological immunological responses have been recognised extensively. In recent years, various studies in both mice and humans have established that Bregs repress inflammatory responses, predominantly through the secretion of interleukin 10 (IL-10) [9]. The regulatory influences of Bregs have been reported in numerous models of autoimmune diseases, inflammation, transplantation reactions, and anti-tumour immunity. Bregs can originate from a distinct B cell subpopulation [10]. Bregs have been determined to act as a suppressive function in the immune system. Bregs can inhibit other immune cells through cytokine production and antigen presentation, which gives them a function in the pathogenesis of cancers and autoimmune diseases [11]. Analysing the B cell subpopulation is a significant area of research due to its therapeutic relevance and role in the immunological response.
Bregs: evidence and origin
The concept of Bregs and a B-cell suppressive event was first noted in 1974, during delayed-type hypersensitivity reactions in guinea pigs [12, 13]. However, it took two decades until the concept of “suppressor B cells” received attention again. Added proof of this B cell modulatory phenotype was evaluated in 1996, which was observed in a murine autoimmune model of experimental autoimmune encephalitis (EAE) where the deficiency of B cells aggravated the inflammation [14]. Then in 1997, it was observed in chronic colitis where the disease worsened in the B-cell deficiency group, suggesting their repressive role in inflammation [15]. The presence of regulatory B cells (Bregs) was confirmed afterwards in 2000 using the term “regulatory B cell”, which described its repressive capability in inflammatory bowel disease [16]. Bregs were reintroduced as an IL-10-producing B cell subpopulation in 2002 [17]. Many attempts have been made in several studies, including mice [18–34] and humans [26, 35–38], at phenotypic identification and characterisation.
Significance statement
Bregs are critical in maintaining self-tolerance [39]. The essentiality of human Bregs in the upholding of immune homeostasis originates from various immune-associated pathologies, such as cancers, autoimmune diseases, and chronic infections that are frequently related to abnormalities in Breg numbers or function [40]. Moreover, to be more specific clinically, such as the role of Bregs in autoimmunity, defects in numbers and functions have been reported in multiple autoimmune diseases, including multiple sclerosis (MS), myasthenia gravis (MG), systemic lupus erythematosus (SLE), rheumatoid arthritis (RA) and psoriasis [35, 41–45]. Inverse correlations were found between Breg numbers and functions, and disease severity status measured using severity scales, e.g, the Multiple Sclerosis Severity Score (MSSS), the Quantitative Myasthenia Gravis score (QMG), the Systemic Lupus Erythematosus Disease Activity Index (SLEDAI), the Rheumatoid Arthritis Severity Scale (RASS), the Psoriasis Area and Severity Index (PASI), etc. These inverse correlations may be due to the loss of immune suppression precipitated by Bregs, giving rise to inflammation, or the reduced Breg numbers and function are a consequence of chronic inflammation. Even though a modulatory function of B cells is well established, more research is required to learn more about their phenotypic identification and characterisation [46]. Knowing the roles of B cells and having the capability to trace an increase or decrease in B cell subpopulation levels can anticipate a useful method for understanding the immunological responses to certain diseases [47]. A constant attempt to perceive Breg biology in healthy controls will yield new possibilities to establish Breg immunotherapy [40].
B cells play a pivotal role in several autoimmune diseases, including patients with MS [48]. Therapeutic interventions targeting B cells have been a useful approach in MS, such as total B cell depletion therapy. The studies describing total B cell depletion therapy using ocrelizumab and rituximab have proven the clinical efficacy in MS [49–51]. B cell depletion might be improved for the treatment of MS, through an approach to deplete subsets of B cells selectively [52], such as in vivo depletion of Bregs. Specific subsets of B cells would give increased advantages over current total B cell depletion therapies [40]. Other therapeutic interventions target Bregs to develop Breg immunotherapy in MS, by B cell isolation of patient-derived PBMCs and in vitro Breg expansion, then adoptive transfer into the patient could suppress inflammation, or in vivo modulation to expand Bregs [40]. These could offer advanced perspectives for therapy of patients with MS.
Material and methods
Materials and equipment
Blood sample with anticoagulation (heparin), Ficoll, phosphate buffered saline (PBS), buffer (ultra-pure water – 1000 ml, NaCl – 8 g, KCl – 0.2 g, Na2HPO4 × 12 H2O – 3.58 g, KH2PO4 – 0.27 g, EDTA – 0.74 g, 0.5% BSA – 5 g), fetal bovine serum (FBS), RPMI-1640 medium, 1% penicillin/streptomycin/amphotericin B mixture, B cell isolation kit II, MS column and miniMACS separator, human IL-10 and TGF-β1 ELISA kit, standard ELISA microplate reader, PerCP CD19, FITC CD5 and PE CD1d antibodies, flow cytometer, water bath (37ºC), refrigerator, cell culture flasks, and dishes, centrifuges, centrifuge tubes, pipettes, haemocytometer.
Ethical consideration and approval, informed consent
The research was performed with the approval of the institutional review board (IRB) of the Hubei University of Medicine, Shiyan, Hubei 442000, China; and the project was evaluated and approved by the assessment panel. The purpose of this research was explained to the participants, and when the oral consent was understood and agreed, in front of a nurse, a consent form was provided in English and Chinese. This process was done to obtain the informed consent as well as the legal identification. The individuals who gave consent were included in the project.
Data collection
We involved all participants from Taihe Hospital of Hubei University of Medicine. We obtained 20 ml blood samples from 40 healthy controls, aged between 18 and 55 years of age. These participants did not receive any medication for cancers or autoimmune diseases.
Preparation and storage of PBMCs
First of all, a human peripheral blood sample was obtained in 20 ml disposable syringes and anticoagulation (heparin) was added. It was then gently diluted in PBS (Good Bio, China) and peripheral blood mononuclear cells (PBMCs) were isolated through Ficoll (TBD Sciences, China) gradient centrifugation based on the manufacturer’s protocol. A culture medium (RPMI-1640 medium + 10% FBS + 1% penicillin/streptomycin/amphotericin B mixture) was added, and then the sample was transferred to two different cell culture flasks using a pipette. Flask 1 was used for isolation and storage of B cells, and flask 2 was used for flow cytometric analysis of PBMCs.
Isolation and storage of B cells
After centrifugation, supernatant was discarded, and cell numbers (PBMCs) were determined using a haemocytometer. Then the B cells were isolated through magnetic separation based on the manufacturer’s protocol utilising an MS column and B cell isolation kit II (Miltenyi Biotec, USA). A culture medium (RPMI-1640 medium + 10% FBS + 1% penicillin/streptomycin/amphotericin B mixture) was added and then transferred to a cell culture flask using a pipette. The flask was used for flow cytometric analysis of B cells and ELISA assays.
Cell staining and flow cytometric analysis
After centrifugation, supernatant was discarded, and cell numbers (PBMCs or B cells) were determined using a haemocytometer. Then the cells were resuspended in buffer and cells were surface stained with CD19, CD5, and CD1d antibodies (BD Biosciences, USA). The cells were then incubated in a refrigerator at 4°C for 30 min, followed by two washing steps to remove any residual antibody from the cell suspension. The samples were interpreted using the flow cytometer BD FACSCanto II (BD Biosciences, USA), and the data were analysed by BD FACSDiva 8.0.1 software.
ELISA
Enzyme-linked immunosorbent assay (ELISA) was performed using a human IL-10 and TGF-β1 ELISA kit (Neobioscience, China) based on the manufacturer’s protocol. The absorbency was assessed by a microplate reader (Thermo Scientific, Finland). After completing the experiments, remaining reagents were put back in a refrigerator for temperature restoration.
Results
Phenotypic Identification of CD19+CD5+CD1d+ Bregs
The CD19+CD5+CD1d+ Bregs were phenotypically identified using specific CD markers: CD19, CD5, CD1d which are the key markers for identifying Bregs [17, 41, 53–56]. Breg cell subpopulations were identified by double positive staining of CD5+CD1d+ Bregs from PBMCs and B cells (Figure 1). This novel CD19+CD5+CD1d+ Breg subpopulation in human peripheral blood was represented (n = 40; 19.27 ±1.52) from PBMCs (n = 40; 33.32 ±2.95) from isolated B cells accordingly. These mean values of Bregs identified here can be referred to as the normal ranges that are responsible for maintaining a dynamic balance through a certain level in healthy controls. Any alterations to the mean values may indicate marked defects.
Figure 1
Analysis of CD19+CD5+CD1d+ Breg expression using flow cytometry from PBMCs and B cells in human peripheral blood. A1, B1, C1 – without staining of an antibody – shows forward scatter (FSC-A) measures cell size and side scatter (SSC-A) measures cell granularity; counting beads (black, red and green) and B cells gates are depicted; A2, B2, B3 – PBMCs with staining of CD19, CD5, CD1d antibody – B cells fating revealed a CD19+ population (green) and a CD19– population (red); A3, B3, C3 – B cells with staining of CD19, CD5, CD1d antibody – CD19+ B cells gating revealed CD5+CD1d+ (Q2) population; Bregs are defined as CD19+CD5+CD1d+
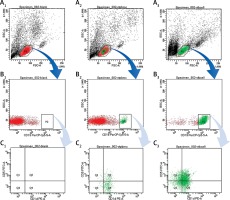
Characterisation of phenotypically identified CD19+CD5+CD1d+ Bregs
The characterisation of phenotypically identified CD19+CD5+CD1d+ Bregs was observed through ELISA assays using human IL-10 and TGF-β1 ELISA kits based on the manufacturer’s protocol. The IL-10 production represents (n = 40; 0.56 ±0.08), and TGF-β1 production represents (n = 40; 0.90 ±0.12) by these phenotypically identified CD19+CD5+CD1d+ Bregs. These mean values of cytokine production can be referred to as the functional properties of Bregs when maintaining a dynamic balance through a certain level in healthy controls. Alterations may disrupt the normal regulatory function.
Discussion
During immunoreaction, B cells manifested positive and negative modulatory influences [57]. The modulatory function of B cells in autoimmune diseases was demonstrated in 1996 in EAE [58]. Bregs’ (B10 cells) existence was confirmed after that by others [58, 59]; B cell subpopulations were found to be capable of influencing immunological tolerance [22, 28, 57, 58, 60, 61]. Bregs expressed a reduced and impaired role in most of the studies. The term “Bregs” was introduced by Matsushita, who established Bregs as an IL-10 secreting B cell subpopulation in 2002 [62]. Bregs carried out diverse mechanisms to regulate immunological responses and aimed for numerous unique immune cell types, such as DCs [63] and macrophages [64] as well as T helper (Th1 and Th2) cells [65]. It was found that Bregs were effective in repressing the expansion of CD4+CD25– T cells [66]. According to the present state of affairs concerning the phenotypes and markers of human Bregs [17, 67–70], they exhibit some of the same phenotypic properties as formerly defined markers in mice [35, 37]. In this study, we phenotypically identified Bregs using specific CD markers by double positive staining of CD5+CD1d+ Bregs in human peripheral blood. In most of the previous studies, Bregs were identified from PBMCs [17, 26, 35–38, 40–45, 53–56, 67–72]. In this study, we used both techniques which include Breg identification from PBMCs and isolated B cells. We observed that the percentage of Bregs was higher if identified from isolated B cells than from PBMCs. Therefore, the identification of isolated B cells provides greater specificity.
Nonetheless, of the variety of markers used to identify Bregs, the protective effects of Bregs are dependent on IL-10 [17, 37, 71, 72], a potent deactivator which limits the potency and time of inflammatory responses. Consequently, IL-10 secretion is still an essential criterion in the identification of Bregs. The leading role of IL-10 is to repress excessive inflammation and control homeostasis via various mechanisms. This mechanism involves hindering antigen presentation and co-stimulatory molecules CD80, CD86, and MHC class II expression through DCs and macrophages. The suppression of excessive inflammation and maintenance of homeostasis can also be regulated by inhibiting cytokine release by T cells in immunological responses and supporting Treg differentiation [73]. While IL-10 secretion remains the hallmark of Bregs’ negative modulatory role, other independent repressive mechanisms, e.g. involving TGF-β1, have been revealed in recent years. Secretion of TGF-β1 by B cells give rise to the support of Treg development in transplant tolerance, diabetes, allergic diseases, and colitis [74]. In this study, we also observed, through ELISA assays, that these novel CD19+CD5+CD1d+ Bregs produce anti-inflammatory cytokines IL-10 and TGF-β1 (see Figure 2 for Breg cell phenotype and character diagram based on the current state of knowledge and this study).
Figure 2
Breg cell phenotype and character diagram based on the current state of knowledge. B cells express clonally distributed antigen presenting receptors (BCRs) that bind to one particular antigen. The mature B cell expresses both immunoglobulin M (IgM) and immunoglobulin D (IgD) [30, 79, 80]. A mature B cell can differentiate into several types of B cells, the most common being plasma and memory B cells [81, 82]. Bregs (also called B10 cells due to their IL-10 expression ability) produce anti-inflammatory cytokines IL-10 and TGF-β1, shown in the circled area (drawn from the current state of knowledge of Bregs and the conclusion of this study)
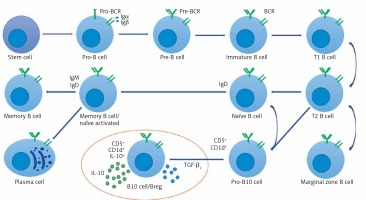
Interestingly, recent research suggests that Bregs can also directly approach the central nervous system (CNS) [31]. In addition, people living with type 1 diabetes express the lowest frequency of B10 cells in comparison with type 2 diabetes, adult latent autoimmune diabetes, and controls [75]. In SLE, Bregs are emerging as an essential player at the time of disease instigation. Adoptive shifting of splenic CD5+CD1dhi Bregs from wild-type mice expanded the time of endurance of CD19–/–mice in this lupus model [76]. In humans, SLE patients showed indistinguishable frequencies of circulating CD24hiCD38hi B cells, but had a remarkably lower IL-10+ proportion in comparison with controls [77]. Rheumatoid arthritis studies have also implied that Bregs are responsible for Tregs’ initial inducement [78]. In one of our recent studies, we identified a lower frequency of CD19+CD5+CD1d+ Bregs in MG and lower cytokine secretion compared to healthy controls. Based on QMG scoring techniques we have also observed that the lower the cytokine levels are, the more clinical symptoms the patient has [41]. Thus, the detailed protocol and methods presented here are extremely valuable and should be able to facilitate progress in research on various autoimmune diseases, cancer, inflammation, and immunologic responses.
In conclusion, Bregs are a significant area of research due to their immune regulatory function in the immunological responses through their potential cytokine production. Through flow cytometric analysis and ELISA assays of B cells of the human peripheral blood sample, either from PBMCs or isolated B cells, the regulatory role may be observed. The healthy controls can be compared with particular autoimmune diseases, inflammation, cancer, and immunologic responses to find out whether Breg alteration and/or cytokine production is altered in these disorders or conditions. If the alteration of Bregs and cytokine production is significant along with the clinical correlation, a further in vitro study can be conducted such as PBMC or B cell culture with exposure to certain drugs. If cytokine production improves, then an in vivo study can be initiated.