Infection by the novel coronavirus SARS-CoV-2 produces a wide spectrum of clinical patterns, which are generating debate in the scientific community. Efforts to describe the clinical characteristics of this disease are made harder by the current pandemic situation in which most countries are either being heavily hit or are slowly recovering after enduring hard months of battle. In particular, many authors have tried to describe different phenotypes of COVID-19-associated acute respiratory distress syndrome (ARDS) (C-ARDS) to individualize treatment and respiratory management [1, 2], but mortality of mechanically ventilated COVID-19 patients remains very high and was estimated to be around 45%, although some studies have reported slightly lower figures [3–5].
The host immune response to infection by SARS-CoV-2 plays a key role in COVID-19 and has been the target of many therapies for the disease; moreover, it may influence the mechanical characteristics of the lungs during the course of the disease. This is due to the inability of the lung to repair itself following sustained injury, which results in persistent inflammatory stimulus and elevation of cytokines, which predicts poor outcome in ARDS [6]. Lung fibrosis is a known consequence of persistent ARDS after a long course of mechanical ventilation [7, 8], and it is associated with inflammatory dysregulation and extremely high mortality [7, 9]. However, few studies have presented data regarding the respiratory mechanics that patients present after a long course of mechanical ventilation for ARDS or for C-ARDS [10–13]. Recently the respiratory mechanics of patients with lung fibrosis were described with the paradigm of the “squishy ball” [14, 15]. This is a respiratory pattern that can often be seen in the later stages of persistent ARDS after a course of mechanical ventilation. In this study we reviewed the respiratory mechanics of patients with C-ARDS and evaluated the incidence of a pattern of respiratory mechanics compatible with restrictive or fibrotic disease – the “squishy ball” [14]. We investigated risk factors for the development of this respiratory pattern, and its association with cytokine production and death.
METHODS
Approval for this study was provided by the Ethical Committee CEI (Ethical Committee Nº 224/20), who waived the need for informed consent.
We conducted a retrospective review of the patient data originating from the electronic information system of the hospital. We included patients with positive SARS-CoV-2 PCR in nasal swab or bronchoalveolar aspirate, age > 18 years, and hospitalization in an ICU. Patients hospitalized between 1 March and 30 April 2020 were included in the study. No sample size calculations were performed.
All patients were treated following the hospital protocol for COVID-19 and its updated versions, which were published throughout the outbreak following newly acquired evidence from clinical trials. The protocol included the administration of hydroxychloroquine (loading dose 400 mg every 12 h for 1 day and 200 mg every 12 h for an additio-nal 4 days), lopinavir/ritonavir (400/100 mg every 12 h for 7 days), azithromycin (250 mg per day for 5 days), and low-dose methylprednisolone after admission to the ICU (1 mg kg-1 per day for 5 days tapering to 0.5 mg kg-1 per day for 5 days). High-dose steroid pulses (> 2 boluses of ≥ 250 mg methylprednisolone) were discretionarily used by some physicians in hospital wards prior to ICU admission. Tocilizumab was used (600 mg IV if > 75 kg, 400 mg if ≤ 75 kg) when plasma IL-6 was > 40 pg mL-1 and PaO2/FiO2 ratio < 200. Lungs were ventilated following a protective ventilation strategy aiming at a tidal volume ≤ 8 mL kg-1 (ideal body weight), plateau pressure < 30 cm H2O and driving pressure ≤ 15 cm H2O. Neuromuscular blockade, prone positioning (PP), and recruitment manoeuvres (RM) were also included in a protocolized approach to ventilatory treatment. Neuromuscular blockade was used only if PaO2/FiO2 ratio < 150 and patient/ventilator dyssynchrony was present. Prone positioning was indicated when PaO2/FiO2 ratio < 150 for more than 12 hours of controlled mechanical ventilation, and cycles of at least 16 hours of prone positioning were applied, which could be repeated after 4 hours of supine ventilation if criteria were met. Finally, the use of recruitment manoeuvres was restricted to cases of refractory hypoxaemia not responding to ordinary ventilatory treatment.
The data on respiratory mechanics was evaluated for each patient daily. We observed a pattern of very low compliance (< 25 mL cm H2O–1), no response to PEEP trial, and low optimal PEEP level of < 6 cm H2O (optimal PEEP being the PEEP level that achieved the maximal static compliance), and no response to prone positioning in patients who required a prolonged course of invasive mechanical ventilation. We referred to this pattern as “restrictive” [14] and evaluated factors correlated with the development of this respiratory pattern.
Four clinical diagnoses were defined to assess the causes of death: (1) refractory respiratory failure, (2) pulmonary embolism (diagnosed by CT scan or clinical signs of right ventricular failure by echocardiography), (3) septic shock, and (4) other causes (haemorrhage, neurological disorders).
Plasma levels of TNF-a and interleukins (IL) were monitored to assess changes in the immune response and help define the best therapeutic strategy. Serum cytokines were quantified using the BD Cytometric Bead Array (CBA) Human Inflammatory Cytokines Kit (BD Biosciences, Franklin Lakes, NJ, USA). Serum samples were mixed with beads coated with capture antibodies specific for TNF-a, IL-1b, IL-6, IL-8, IL-10, and IL-12p70 and then conducted according to the manufacturer’s instructions. Beads were analysed by flow cytometry using a FACSCanto II cytometer. Analysis was performed using FCAO Array Software v3.0 (BD Biosciences).
Statistical analysis
Data are presented as median (IQR) for continuous variables because a normal distribution could not be assumed as per Shapiro-Wilks normality tests. Categorical variables are represented as n (%). Univariable analyses for continuous variables were performed using Wilcoxon rank sum tests, and chi-squared tests were used for categorical variables. We analysed the association between the restrictive mechanical pattern and steroid pulses after adjusting for severity (APACHE II) and age using a multivariable logistic regression model. A multilevel linear mixed model was used to estimate the association between the restrictive pattern and (logarithm) IL-6 overtime. The model included fixed effects for the restrictive pattern, time, and the interaction term restrictive pattern x time and random intercepts for patients. Statistical significance was defined as a P-value ≤ 0.05. Statistical analysis was performed with Stata version 16 (StataCorp LP, College Station, TX, USA).
RESULTS
Between 2 March and 29 April we attended to 141 patients in our ICUs, of whom 52 (36.8%) died before leaving the ICU. The characteristics of the population are described in Table 1. At admission 84 patients (60%) had severe ARDS; all patients in the population were treated with invasive mechanical ventilation. Thirty-four patients (65%) died with refractory respiratory failure and persistent hypoxaemia (PaO2/FiO2 < 100). Thirty-one patients presented during mechanical ventilation with a restrictive pattern of respiratory mechanics. The appearance of the restrictive pattern of respiratory mechanics occurred after a median of 10 days of mechanical ventilation (IQR 6–14) and a median of 23 days after the onset of COVID-19 symptoms (IQR 14–26). This pattern of respiratory mechanics was associated with severely impaired gas exchange and a PaO2/FiO2 ratio < 150. Twenty-nine of these patients died (56% of the deceased). Data regarding the evolution of the respiratory mechanics shown in mechanically ventilated patients over time are displayed in the Supplementary Table.
TABLE 1
Characteristics of the population
Other diagnoses related to death were pulmonary embolism (n = 7, 13%), septic shock (n = 17, 33%), and other causes (n = 10, 19%), with some patients combining at least 2 of these diagnoses. Thus, patients who developed a restrictive respiratory pattern during the disease were very likely to die with severe hypoxaemia. Severe lung injury was observed in the first autopsy performed at our hospital, showing diffuse alveolar damage in the advanced organization/proliferation phase together with areas of well-developed fibrosis (Figure 1) [16].
FIGURE 1
Histological section of the lung showing severe reduction of air spaces with septal thickening and fibrosis
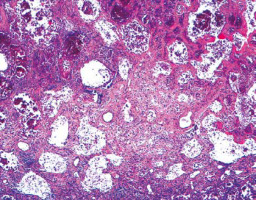
We analysed several risk factors that are potentially associated with the development of this pattern. In a univariate model, age, severity of disease at ICU admission, and the use of steroid pulses showed a significant association with the development of this pattern of respiratory mechanics (Table 2).
TABLE 2
Risk factors for developing a restrictive pattern. Obesity was defined as a body mass index > 30. Steroid pulses included the administration of more than 2 doses > 250 mg of methylprednisolone for more than one day. In brackets: percentage or range
In a multivariate model we analysed the association of high-dose steroid pulses and the pulmonary restrictive pattern adjusted by age and APACHE II. After adjusting, age (OR = 1.06; 95% CI: 1.01–1.12; P = 0.029) and the administration of steroid pulses were associated with higher risk (OR = 2.7; 95% CI: 1.1–6.8; P = 0.03) (Table 2).
Cytokines (IL-1b, IL-6, IL-8, IL-10, IL-12, and TNF-a) were measured routinely in the plasma of patients hospitalized in the ICU. Logarithmic values of IL-6 were significantly higher and increased significantly over time in patients who developed a restrictive pattern of respiratory mechanics (P < 0.001), but they appeared to slightly decrease in patients who did not (P = 0.084) (Figure 2).
DISCUSSION
This study evaluated the incidence and impact on clinical outcome of a ventilatory pattern compatible with acquired restrictive pulmonary disease in COVID-19 mechanically ventilated patients. We showed that patients who present this ventilatory pattern have a high incidence of mortality, as well as a strong activation of the innate immune system.
To our knowledge, this is the first study to describe this type of ventilatory pattern in late COVID-19- associated ARDS. We observed the emergence of a restrictive pattern in the mechanical properties of the lungs of 31 patients, of whom 29 (93%) subsequently died, constituting 56% of the overall mortality registered in our study. Early reports in ARDS have shown a similar pattern of restrictive disease to be present in the late stages of the syndrome [17]. However, later studies during the era of lung protective ventilation did not show the same results [18]. The high incidence of this type of respiratory pattern is concerning, and it could be related to ventilator-induced lung injury, as was probably the case in the earlier reports of late ARDS lung mechanics before the era of lung protective ventilation [18]. However, it must be underlined that the patients included in this study were all treated following strict lung protective strategies in a tertiary hospital that cares for a high volume of ARDS patients every year. The mechanical properties of the lungs of patients who developed a restrictive pattern as per our criteria were similar to the ones shown by ARDS patients with fibrotic lungs (due to idiopathic pulmonary fibrosis) published in the literature [14, 15]. In particular, the application of incremental PEEP produced an increase in elastance and therefore an increase in parenchymal stress [14, 15]. In our experience, in the initial phase after intubation, COVID-19 patients are usually characterized by a positive response to RM and PP. However, despite lung protection, a remarkable reduction in lung compliance gradually appears in some ICU patients, usually after 10 days of disease. Several studies in C-ARDS and ARDS by other aetiologies have described lung mechanics to be highly variable and not related with the impairment of gas exchange [10–13, 19–21]. In some cases, lung compliance on the first day of mechanical ventilation was associated with mortality [20, 21]; however, the association was not as clear in other reports [19]. In particular, lung recruitability was found to be high in the majority of patients in the first hours of mechanical ventilation, and to follow a variable pattern in the following days of treatment [22]. The parameters of respiratory mechanics over time show a progressive worsening with reduced compliance associated with stable PaO2/FiO2 ratio and worsening ventilatory ratio; however, none of these studies provides respiratory mechanics parameters beyond day 7 of mechanical ventilation, and there is no description of the emergence of specific patterns in the study population [12]. The pattern of respiratory mechanics analysed in this study appeared after 10 days (IQR 6–14) of protective mechanical ventilation, and it is compatible with clinically relevant lung fibrosis. None of the patients included in this study underwent lung biopsy; therefore, the clinical suspicion of lung fibrosis given by the restrictive pattern of respiratory mechanics cannot be confirmed. However, in all the autopsies performed on COVID-19 ICU patients in our centre, areas of well-developed pulmonary fibrosis were described [16]. Several reports of pulmonary fibrosis following severe COVID-19 have been published recently and confirm the high prevalence of this finding [23, 24], which is associated with the severity of the viral pneumonia [25]. McGroder et al. [23] found significant alteration in the post-discharge CT scans of 72% of C-ARDS survivors who had been mechanically ventilated, whereas Zou et al. [24] found them in 100% of ICU survivors. Moreover, in the study by Zou et al. [24] there was a clear association between fibrotic changes and the level of inflammatory markers and notably IL-6 during the acute phase of the disease. These findings coincide with previous observations showing that the development of pulmonary fibrosis is common after a SARS coronavirus infection [26]. Fibrosis in acute lung injury is related to complex biological reactions where inflammatory cytokines and neutrophils act as primers [27]. Sequential exudative (early) and fibroproliferative (late) phases defined although proliferation have been observed early in ARDS [28]. This pathogenic mechanism has been thoroughly studied in the last decades, but no successful treatment has been found. Ventilator-induced lung injury (VILI) is associated with fibrosis, and the implementation of protective ventilation leads to a reduction in mortality [29].
We have analysed several factors that might have an influence on the restrictive progression. Both sepsis [30] and obesity [31] involve a degree of inflammatory dysregulation despite being 2 different processes causing distinct alteration in immune physiology. In our analysis neither was associated with the development of clinical pulmonary restrictive features. A predominant role of the innate over the adaptive immunity is probably related to the persistent release of mediators that finally favour the fibroproliferative phase in lung injury. The blockade of the adaptive response may be secondary to immunosenescence (T-cell exhaustion), lymphopaenia secondary to viral infection, or immunosuppression [32]. Thus, immunosenescence in older patients may explain the greater risk of developing a restrictive pulmonary pattern associated with age [23]. High-dose steroid pulses (on top of the normal treatment with steroids given to ARDS patients) were also related to the development of this pattern. A possible mechanism to explain this finding is an excess of generalized immunosuppression affecting the adaptive response, which induces a rebound of the innate immune response [32]. However, it is difficult to interpret these data because the criteria for the use of steroid pulses were not clearly defined. On the other hand, it must be underlined that steroid pulses were in all cases administered in hospital wards and before ICU admission was needed. Moreover, a recent study has demonstrated different patterns of response in C-ARDS patients to therapy with corticosteroids, indicating possible harm from these drugs in specific subpopulations [33].
Patients who developed a clinical pulmonary restrictive pattern presented a different immune response compared to other COVID-19 ARDS patients, as described by cytokine production. In fact, they showed a dramatic increase in plasma levels of IL-6 but not in the other cytokines measured (IL-1b, IL-8, IL-10, IL-12 and TNF-a). This suggests that IL-6 is the main inflammatory cytokine in SARS-CoV-2 associated ARDS and its late fibroproliferative stages. Persistent activation of the innate immune system, suggested by high IL-6 values, can contribute to the development of pulmonary fibrosis and highlights the importance of an early control of innate cell inflammation because it can derive in the production of mediators (e.g. oxygen reactive species or proteases), which may induce lung damage resulting in fibrosis. The reason for the persistent inflammation is unknown.
ARDS and idiopathic pulmonary fibrosis acute exacerbations (IPF-AE) share many clinical and histological features. IPF-AE may be related to intrinsic biological dysfunction of the lung, which makes some individuals more susceptible to external insults such as VILI [9, 14], hyperoxia, and viral infections [34], all of which are present in COVID-19 patients. The restrictive pattern observed in COVID-19 probably shares biological processes with both late ARDS and IPF-AE; thus, we may speculate that previous experience in these scenarios may be useful in the case of COVID-19. Many drugs have been used to prevent or treat fibrosis in ARDS. The use of early low-dose steroids (methylprednisolone 1 mg kg–1 per day or equivalent doses of dexamethasone) has been advocated in ARDS [35, 36] and has shown positive effects in COVID-19-associated ARDS [37]. However, high-dose steroid pulses or late use of low-dose steroids raise concerns because they might increase mortality [38, 39].
There are several limitations to this study. The fact that it is single-centre, retrospective, and includes a relatively low number of cases precludes the generalizability of our findings. Second, we evaluated the respiratory mechanics of patients but did not perform further tests such as lung biopsies or HRCT to confirm the clinical suspicion of lung fibrosis. Moreover, we did not evaluate the smoking status, which has been correlated with severe C-ARDS. In respect to IL-6, the persistence of high levels of this mediator might be influenced by the administration of tocilizumab because the IL-6 receptor blockade can increase or decrease the plasmatic levels of IL-6. However, because the protocol for the use of tocilizumab was common for all patients, this should have been seen in non-restrictive patients as well, because tocilizumab was administered whenever IL-6 levels were raised (as was the case in all the patients analysed). Because IL-6 levels were measured routinely and all our patients were treated according to the same protocol, it is not possible to discern the role of tocilizumab in this regard. Regarding the role of steroid boluses, we must acknowledge the possibility of a survival bias because patients who were given early steroid boluses may have survived the initial stage of the disease to then develop a restrictive pattern later on. The strength of the study is that we have included a homogeneous cohort of patients with ARDS caused by the same pathology and were treated with the same pharmacological and ventilatory protocol during their ICU stay, thus limiting confounding factors based on different therapeutic approaches.
We conclude that COVID-19 patients requiring a prolonged course of mechanical ventilation often develop a restrictive pattern of respiratory mechanics despite the use of protective ventilation strategies. This ventilatory pattern is associated with sustained activation of the innate immunological response, and it has a very high mortality rate. Age and the administration of high-dose steroid pulses prior to ICU admission are the main risk factors associated with the development of this respiratory pattern.