Introduction
The digestive tract is the largest frontline immune organ that faces invasive microorganisms. Each type of immune cell, including 60–70% of total lymphocytes, is distributed in the intestinal tract [1]. It has recently been shown that the immune status determined by the local intestinal pathogens, including intestinal microbiota and parasitic worms, determines the immune condition of the total body, regulates the strength and specificity of the immune reaction, and controls the onset of autoimmune disease [2]. Immune disorder-based colitis in humans, including the widely recognized inflammatory bowel diseases (IBDs) such as ulcerative colitis and Crohn’s disease, are common situations in which immune conditions involving the whole body and the pathogens exposed to the immune system affect each other.
Dextran sulphate sodium (DSS) is frequently used to induce experimental colitis [3, 4]. DSS directly destroys the colon area of gut epithelial cells and disrupts the physical barrier to intestinal bacteria, causing bacteria to invade the subepithelial area, leading to an immune response. Simultaneously, DSS reaches the submucosal area and is captured by antigen-presenting cells (APCs), which stimulate T cells.
Titanium oxide (TiO2) is a photocatalytic inactivator of various materials, including environmental pollutants and microorganisms (viruses, bacteria, etc.) [5, 6]. Most organic materials are disassembled by the action of reactive oxygen species (ROS) that are produced by the catalytic action of TiO2, which converts light energy to decomposition energy. TiO2 was only activated by UV light at ~300 nm wavelength. Therefore, doping technology using metal ions, such as Ag, Fe, and Co, are commonly adopted. Considering its strong bactericidal effect, TiO2 may be useful for the management of IBDs, including colitis, because the intestinal microbiota closely affects the onset of IBD. Although this antibacterial action may be useful for the treatment of colitis, simple TiO2 may be harmful to mammalian host cells, including intestinal epithelial cells, vascular endothelial cells, and immune cells. To improve the targeting of bacteria, TiO2 is coated with hydroxyapatite (HA), which preferentially binds to the bacterial cell wall and prevents direct interaction between TiO2 and host cells [7, 8]. HA is a widely used material in dental care, which has a unique feature of capturing bacteria and acts as a good intervention that regulates the action of TiO2 against its target.
Aim
In this study, we investigated the effect of HA-coated TiO2 (HA-TiO2) on DSS-induced colitis in mice.
Material and methods
Mice and experimental design
Six-week-old female C57BL/6J mice were purchased from SLC Japan (Hamamatsu, Japan) and maintained under stable conditions at 25°C. The mice were divided into 4 groups: 1) normal control; 2) 4.5% DSS in drinking water; 3) 4.5% DSS in drinking water, and fed with 0.5 mg/day HA-TiO2; and 4) 4.5% DSS-treated plus 0.5 mg/day HA-TiO2 fed in dark condition. The mice were kept under fluorescent light at ~800 lx throughout the experiment, except for one group kept in an absolutely dark room throughout the experiment. The experimental design is illustrated in Figure 1.
Figure 1
Design of the experiment. Mice were divided into 4 groups. Mice in the (Normal) group were kept under fluorescent light throughout the 7 days of the experiment and were maintained with usual drinking water. Mice in the (4.5% DSS) group were kept under fluorescent light and were treated with 4.5% dextran sulphate sodium (DSS) in drinking water. Mice in the (DSS + HA-TiO2) group were kept under fluorescent light, treated with 4.5% DSS in drinking water, and were daily fed with 5 mg hydroxyapatite (HA)-coated titanium oxide (TiO2). Mice in the (DSS + HA-TiO2 Dark) group were kept in a dark place throughout the 7 days of the experiment, treated with 4.5% DSS in drinking water, and fed daily with 5 mg HA-coated TiO2. Mice were sacrificed at 7 days after initiation of the experiment and were subjected to the analysis
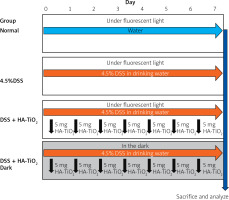
Dispersing hydroxyapatite-coated titanium dioxide particles
TiO2, of which approximately 10% was coated with HA, was obtained from NANOWAVE. Co. Ltd. (Seto, Japan) [9]. Briefly, TiO2 was suspended in a pseudo-body fluid containing a dispersant (sodium polyacrylic acid) at an even density by stirring. Sodium phosphate and calcium chloride solutions were added to prepare the precipitant. The precipitated HA-coated TiO2 was then subjected to a dispersion step. The dispersion was performed using a bead-mill crusher or a jet-mill crusher. The dispersed TiO2 was suspended in distilled water (DW) to achieve a TiO2 concentration of 10% (w/v).
Colitis induction by DSS and administration of HA-TiO2
DSS 5000 (FUJIFILM, Osaka, Japan) was dissolved in drinking water at 4.5% (w/v). The original HA-TiO2 suspension (10%) was diluted 10 times with DW, and 0.5 ml of diluted suspension containing 5 mg HA-TiO2 was forcibly fed to the subjects using plastic feeding tubes (FTP-20-38-50, Instech Laboratories Inc., PA, USA).
Antibodies
Rabbit anti-mouse CD4 (clone 50134) and anti-mouse CD8 (clone 50389) monoclonal antibodies were purchased from Sino Biologicals Inc. (Wayne, PA, USA). Rabbit anti-mouse F4/80 antibody (clone D2S9R) was purchased from Cell Signaling Technology Inc. (Danvers, MA, USA).
Histology
The colon was cut into round slices, fixed with 10% formalin for 24 h, paraffin-embedded, sectioned, deparaffinized, rehydrated, and finally stained with haematoxylin and eosin (H&E). The samples were dehydrated and covered with water-insoluble mounting media and cover glass.
Histological score
Histological scores were blindly scored using H&E-stained colonic tissue sections according to the established scoring system [10]. Points according to the severity of changes in crypt architecture (0–3 pt), inflammatory cell infiltration (0–3 pt), muscle thickening (0–3 pt), goblet cell depletion (0–1 pt), and crypt abscess (0–1 pt) were summed for each mouse.
Immunohistochemistry
Formalin-fixed, paraffin-embedded slides were deparaffinized with xylene and then gradually rehydrated by transferring the slides from 100% EtOH to 70% EtOH. The antigen recovery process was performed by boiling the slides in citrate buffer (pH 6.4) using microwaves. Staining with antibodies was performed using a PolyStain DS KIT purchased from NeoBiotech (Nanterre, France). Briefly, the first primary antibody was diluted 200 times with phosphate-buffered saline (PBS), applied to the slides, incubated in a humidified chamber for 40 min at room temperature, then washed 3 times with PBS-tween20 (PBS-T). The secondary anti-rabbit IgG antibody-alkaline phosphatase polymer was applied and incubated for 20 min, washed with PBS-T, and incubated for 10 min with a mixture of permanent red substrate, activator, and chromogen. After vigorous washing, inactivator A was applied and incubated for 30 min, followed by 5 min of incubation with inactivator B, then washed with PBS-T, incubated with the second primary antibody for 40 min, washed with PBS-T, and incubated with the secondary anti-rabbit IgG antibody, horseradish peroxidase (HRP), for 20 min. After counterstaining with 5-times diluted haematoxylin solution and washing, emerald chromogen was applied to the slides and incubated for 5 min. The slides were then washed and dried at 85°C for 10 min. The stained specimens were fixed with 100% methanol, dehydrated with 100% ethanol and xylene, and mounted with water-insoluble mounting media and cover glass.
Numbers of infiltrated cells
Immunohistochemically stained colon sections were microscopically examined using a 20× objective lens and viewed on a PC display. The number of cells stained with the expected colour was counted for 3 randomly selected fields, and the sum of 2 independent sections for each mouse was calculated.
Intestinal microbiota analysis of mouse faeces
Mouse faeces were obtained from the colon after the mice were sacrificed. DNA was extracted from the samples and amplified for a specific sequence, and each fragment was sequenced. The number of fragments identified as specific bacterial species based on their DNA sequences was analysed. The intestinal microbiota analysis was performed by TechnoSuruga Laboratory Co., Ltd. (Shizuoka, Japan).
Ethics Committee Approval
The authors declare that the research was conducted according to the principles of the Regulations on Animal Experiments in Nagoya University and approved (approval number 31412) by the Centre for Anima Research and Education, Nagoya University Graduate School of Medicine, Japan.
Results
Body weights were calculated daily (Figure 2). Apparent body weight loss was observed 6 days after the initiation of DSS administration. Although body weight loss was also observed in HA-TiO2-fed mice, the loss was significantly lower than that in the DSS-treated mice (Figure 2).
Figure 2
Body weight of mice with DSS-induced colitis. Mice were maintained with usual drinking water (Normal, black line, n = 2), with 4.5% DSS-containing water (4.5% DSS, blue line, n = 6), with 4.5% DSS-containing water fed with 5 mg/day of HA-TiO2 (DSS + HA-TiO2, red line, n = 5), or maintained in the dark with 4.5% DSS in drinking water fed with 5 mg/day HA-TiO2 (DSS + HA-TiO2-Dark, yellow line, n = 5). Mice were kept under fluorescent light at ~800 Lux except for the group maintained in the dark. Averages with error bars of standard deviation are presented as percentages of initial body weight (day 0). Significantly different (*0.01 < p < 0.05, **p < 0.01). Not significantly different (†p > 0.05)
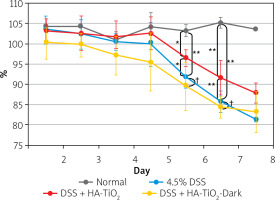
To evaluate the severity of colitis, the colon length was measured when the mice were sacrificed 7 days after the initiation of DSS administration. As shown in Figures 3 A and B, the colon length of DSS-treated mice was shorter than that of untreated mice. This reduction in colon length was significantly improved by the administration of HA-TiO2 (Figure 3 B).
Figure 3
Colon of mouse with DSS-induced colitis. Mice were sacrificed at 7 days after initiation of the experiment. A – Macroscopic appearance of the colon. Note the massive haemorrhage and diarrhoea stool in the DSS colon. Such haemorrhage and diarrhoea disappeared in mice fed with HA-TiO2. B – Colon length. Box and whisker plot showing box as lower and upper quartile and whisker as minimum and maximum values in each group (n = 6 for control, n = 10 for 4.5% DSS, n = 12 for DSS + HA-TiO2, and n = 10 for DSS + HA-TiO2 kept in the dark). Significantly different (*0.01 < p < 0.05, **p < 0.01). Not significantly different (†p > 0.05)
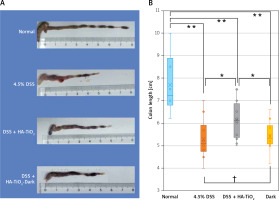
Weight loss was observed in the group of mice kept in the dark throughout the 7 days of the experiment similarly to those treated with DSS without HA-TiO2 feeding (Figure 2). Furthermore, the improvement in colon length by HA-TiO2 feeding was not significant in mice kept in the dark, and the colon length was significantly different between HA-TiO2-fed mice kept in the dark and those exposed to light (Figure 3 B). These observations support the photo-dependent activity of TiO2 for the amelioration of DSS-induced colitis.
Histological examination of the colon was performed using H&E-stained tissue sections. Figure 4 shows the typical pathogenic changes in each of the 4 experimental groups. In all the sections examined, DSS-treated mice without TiO2-administration showed strong signs of inflammation associated with disruption of mucosal epithelium, thickening of both mucosal and submucosal layers, and massive infiltration of inflammatory cells (Figure 4 B). In contrast, the pathological signs in the colons of HA-TiO2-fed mice were dramatically improved, even though they were treated with drinking water containing the same concentration of DSS. In general, the examined sections were mostly intact (Figure 4 C). However, the colons of mice that were fed with HA-TiO2 but were kept in the dark showed pathological images of inflammatory signs similar to those treated with DSS alone (Figure 4 D). These histological findings were confirmed by scoring (Figure 4 E). The histological score was significantly reduced in DSS plus HA-TiO2-treated mice exposed to light compared with that in DSS-treated mice, but not in DSS plus HA-TiO2-treated mice kept in the dark. This result indicates the importance of light on the effects of TiO2. Thus, judging from the changes in body weight, length of the colon, and pathological examination of the colon, it was confirmed that HA-coated TiO2 can prevent more severe progression of DSS-induced acute colitis depending on its photocatalytic activity.
Figure 4
Histological analysis of the colon. Normal (A), 4.5% DSS-treated (B), DSS-treated and HA-TiO2 fed (C), and DSS-treated and HA-TiO2 fed-kept in the dark (D). Mice were sacrificed at day 7, and their colon sections were stained with haematoxylin and eosin. Pictures with typical changes representing each group are shown. All the pictures were taken with a 10× objective lens. E – Histological scores were calculated and the average of 3 mice in each group are shown with error bars of standard deviation. Significantly different (*0.01 < p < 0.05, **p < 0.01). Not significantly different (†p > 0.05)
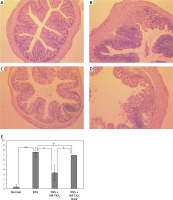
When TiO2 was not appropriately coated with HA, the results were inconclusive (data not shown), implying that coating TiO2 with HA is crucial for its activity.
Macrophages and CD4+ or CDF8+ T cells were detected in the colon tissue by immunohistochemical analysis using anti-F4/80 monoclonal antibody (mAb) and anti-CD4/CD8 mAbs to detect macrophages and T cells, respectively. As shown in Figure 5, a considerable number of macrophages were observed in the colon tissue of mice with DSS-induced colitis (Figure 5 B), whereas they mostly disappeared in the colon tissue of mice treated with HA-coated TiO2 (Figure 5 C). Macrophages re-appeared in the colon tissue of mice who were treated with HA-coated TiO2 but remained in the dark (Figure 5 D). The number of infiltrated macrophages was significantly reduced in DSS plus HA-TiO2-treated mice exposed to light, compared with that in DSS-treated mice, but not in DSS plus HA-TiO2-treated mice kept in the dark (Figure 5 E). T cells were examined using the CD4 and CD8 markers. Massive inflammatory cell infiltration was observed in the colon of DSS-treated mice, and this infiltrate was abundant in CD4+ and CD8+ T cells (Figure 6 B). Such T-cell infiltrates were absent from the colon of HA-TiO2-treated mice exposed to fluorescent light (Figure 6 C), but they were observed in mice kept in the dark (Figure 6 D). Both the numbers of infiltrated CD4+ cells and CD8+ cells were significantly reduced in DSS plus HA-TiO2-treated mice exposed to light compared with that in DSS-treated mice but not in DSS plus HA-TiO2-treated mice kept in the dark (Figure 6 E). Thus, macrophages and T cells appeared to be involved in the process of DSS-induced colitis and its amelioration by HA-TiO2.
Figure 5
Immunohistochemical analysis detecting macrophage-specific molecule F4/80. Normal control (A), 4.5% DSS-treated (B), DSS-treated and HA-coated TiO2 fed (C), DSS-treated and HA-TiO2 fed-kept in the dark (D). F4/80-expressing macrophages are shown by Permanent Red staining and indicated by red arrows. Pictures with typical changes representing each group are shown. All the pictures were taken with a 20× objective lens. E – Numbers of macrophages were calculated, and the average of 3 mice in each group are shown with error bars of standard deviation. Significantly different (*0.01 < p < 0.05, **p < 0.01). Not significantly different (†p > 0.05)
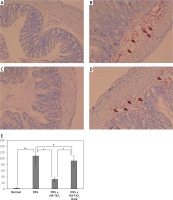
Figure 6
Immunohistochemical analysis of the colon for CD4 and CD8 expression. CD8 was stained with Permanent Red and CD4 was stained with Emerald. Normal colon of a control mouse (A), 4.5% DSS-treated colon (B), DSS-treated and HA-TiO2-fed colon (C). DSS-treated and HA-TiO2 fed colon kept in the dark (D). Pictures with typical changes representing each group are shown. CD8+ cells are stained with Permanent Red and indicated by red arrows. CD4+ cells are stained with Emerald and indicated by green arrows. All the pictures were taken with a 40× objective lens. E – Numbers of CD4+ cells and CD8+ cells were calculated, and the average of 3 mice in each group are shown with error bars of standard deviation. Significantly different (*0.01 < p < 0.05, **p < 0.01). Not significantly different (†p > 0.05)
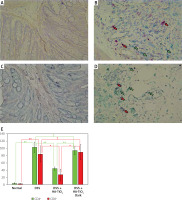
Intestinal microbiota were analysed for faeces obtained from DSS-treated mice, either with or without HA-coated TiO2. Figure 7 shows obvious changes in the species of bacteria residing in the mouse colon. Analysis of the intestinal microbiota revealed that the components of the bacteria dramatically changed after DSS treatment. In brief, Lactobacillus, Prevotella, and Clostridium cluster XVIII were relatively decreased, while Clostridium subcluster XIVa and Clostridium cluster XI were increased. Among these changes, Lactobacillus, Prevotella, and Clostridium XVIII, which were decreased by DSS treatment, were all unchanged by HA-TiO2 treatment. In contrast, Clostridium subclusters XIVa and XI, which are increased by DSS treatment, were decreased by HA-TiO2 treatment. A decrease in Clostridium cluster XI, which is possibly linked with the increase in Bacteroides, may be crucial for the activity of HA-TiO2.
Discussion
Our results are in contrast to previous results for TiO2 nanoparticles that exacerbated DSS-induced colitis [11–13]. The different results may indicate a fundamental difference in the relationship between the TiO2 nanoparticles and HA-coated TiO2. As assumed before our work, simple TiO2 nanoparticles may directly interact with mouse intestinal epithelial cells (IECs), macrophages, and dendritic cells, performing photocatalytic activity on these cells [14]. In the case of HA-coated TiO2, direct interaction of TiO2 with mouse cells could be avoided with HA. To succeed in the experiment described in this study, there are 2 important points to consider. First, TiO2 was coated with HA. The coating process of TiO2 by HA is crucial because incomplete coating processes or HA alone do not show colitis-suppressing activity (data not shown). In addition to the above-mentioned protective activity of HA in mouse cells, HA preferentially binds to the bacterial cell wall and possibly maintains its position in the intestinal lumen. Secondly, light is required for the activity of TiO2. The mice were exposed to fluorescent light. Because we used Fe-doped HA-TiO2, TiO2 is activated not only by UV light but also by a wide range of visible light. Fluorescent light, including near infrared light, can infiltrate the skin, abdominal wall, and intestinal wall of mice, activating TiO2 in the intestinal lumen [15]. We performed an experiment in which mice were kept in the dark without any exposure to light for 7 days and showed that HA-coated TiO2 did not affect DSS-induced colitis. Although this result is important, suggesting the requirement of light for the photocatalytic activity of TiO2, the other conditions of the mice could not be controlled to the same level, leaving the possibility that the results are not validated.
DSS affects innate immunity by influencing macrophages [16, 17]. Recent studies have shown that there are 2 different types of macrophages: one with the usual activity that positively correlates with the strength of the immune response and one that suppresses the immune response. It has been reported that DSS may affect both types of macrophages, thus complicating the results of the immune response in DSS-induced colitis.
DSS also affects acquired immunity [18–20]. In the case of DSS-induced colitis, the cells that infiltrated the swollen submucosal area contained CD4+ and CD8+ T cells, indicating that the acquired immune system was working 7 days after the initiation of the DSS-induced colitis treatment. HA-TiO2 also decreased the number of infiltrated T cells, although it is more likely that HA-TiO2 caused changes in the intestinal microbiota that led to the change in T-cell dynamics rather than HA-TiO2 directly affecting T cells.
We observed a beneficial effect of HA-coated TiO2 on DSS-induced colitis. This result may directly lead to its application in the management of colitis. For example, feeding this reagent to livestock animals may be useful for preventative purposes.