Introduction
Orthotopic heart transplantation (OHT) is the standard-of-care for end-stage heart disease of any cause. According to the data from the International Society for Heart and Lung Transplantation (ISHLT), in 2015 more than 5000 heart transplantations were conducted worldwide, setting an all-time record in OHT volume across the globe [1]. Despite significant improvement in survival of patients after OHT, the overall mortality remains relatively high, with median survival oscillating around 10 years after transplantation [1]. One of the most important causes of death in this group of patients is cardiac allograft vasculopathy (CAV), which accounts for up to one in eight deaths in the one year and almost 1 in 3 deaths in the 5 years after transplantation [1]. Its incidence is approximately 30% in the 5 years and near 50% in the 10 years after transplantation [1].
The major strategy of CAV detection supported by the guidelines is coronary angiography performed in consecutive periods after transplantation [2]. However, angiographically, CAV is usually detected once it is highly progressed. Intracoronary imaging in the form of intravascular ultrasound (IVUS) and optical coherent tomography (OCT) allow for more sensitive and specific detection of pathologies in the coronary arteries, but their clinical utility in detection and surveillance of patients with CAV still requires close attention. In light of the currently available pharmacotherapy, there is no ascertained treatment for CAV, hence its early detection does not necessarily result in better outcome of treatment.
The aim of this review is to assess the clinical value of optical coherence tomography and other intravascular imaging modalities in patients after OHT.
Pathophysiology
As derived from the pathological findings, CAV is characterised by an excessive proliferation of arterial smooth muscle cells, and deposition of free lipid particles and inflammatory cells. There are numerous established risk factors of CAV, and although its aetiology seems to be primarily immunological, nonimmunological factors may also play an important role in its development [3–5].
From transplantation, endothelial cells of cardiac allograft are subjected to recognition by the recipient’s T cells. Activation of T cells triggers an immunological response cascade, including secretion of cytokines, endothelial cell activation, expression of adhesion molecules, and further recruitment of inflammatory cells, leading to the proliferation of both endothelial and smooth muscle cells [6–8]. There is also evidence suggesting the contribution of the humoral response in the development of CAV because the generation of specific HLA-directed antibodies is an independent risk factor of a dismal outcome after OHT [9–12].
The differing results of treatment of patients after OHT with different immunosuppressive modalities support the involvement of inflammatory processes in the development of CAV. In a recent meta-analysis, treatment with mTOR inhibitors (sirolimus/everolimus) was associated with a significant reduction of maximal intimal thickness when compared with calcineurin inhibitor/mycophenolate mofetil; however, at the cost of a higher risk of acute cellular graft rejection [13].
Factors unrelated to immunological stimulation could be divided into modifiable and non-modifiable, with the majority of modifiable risk factors having a significantly higher prevalence in patients after OHT than in the general population [14]. Among them, the most common are disorders of lipid and glycaemic profile. Dyslipidaemias occur in 59–87% and diabetes in 22–37% of patients 1 and 5 years after transplantation, respectively [15]. Both these conditions could be caused by pharmacotherapy taken after OHT. For instance, hyperglycaemia is a frequent adverse effect of steroid administration, while chronic treatment with calcineurin inhibitors, such as cyclosporine or tacrolimus, can lead to hypercholesterolaemia, arterial hypertension, and chronic kidney disease.
The interplay of immunological and nonimmunological factors finally leads to endothelial injury and vascular inflammatory response [16]. Proper endothelial function has been shown to be crucial in the maintenance of vascular tone and prevention of leukocyte adhesion and platelet aggregation [17]. Once the vasoprotective features of endothelial cells diminish, the local vasculature is subjected to increased risk of improper arterial wall relaxation, platelet adhesion, and thrombosis, but most importantly – excessive fibroproliferation and remodelling [16].
As briefly described, the mechanism of CAV development is substantially different from native atherosclerotic coronary artery disease (CAD). The major differences between CAD and CAV are summarised in Table I.
Table I
Angiographic image of CAV
According to the ISHLT nomenclature criteria, the presence and advancement of CAV should be assessed with the combination of coronary angiography imaging and signs of allograft dysfunction, and divided into the following grades: “not significant”, “mild”, “intermediate”, and “severe” (Table II) [18, 19]. Using the definitions established by the ISHLT, the prevalence of any CAV is 7.8% in the first year after OHT and reaches 47% 10 years after transplantation [1]. Although diagnosis and prognosis of CAV based on the angiography performed routinely after OHT is recommended in the ISHLT guidelines, angiographic assessment of CAV possesses considerable limitations, mostly concerning its – sometimes inadequate – sensitivity and specificity [20, 21]. The reasons for this lay in the pathomechanism of CAV, which consists of two phases: first being an early thickening of the intima, and second being late remodelling with arterial lumen constriction [22]. In the early phase of CAV, an arterial lumen is not necessarily obstructed; therefore, it is sometimes impossible to evaluate the presence of this stage of the disease only with angiography. Furthermore, because the disease often occurs in the whole coronary tree, affecting the whole longitudinal dimension of the arteries, and the prevalence of focal stenoses is significantly limited, the number of available reference segments used for comparison is also significantly reduced.
Table II
ISHLT recommended nomenclature for cardiac allograft vasculopathy. Adapted from the 2010 ISHLT consensus statement for recommended nomenclature of CAV [18]
Therefore, there is a growing need for the introduction of more sensitive methods of CAV assessment, such as intravascular imaging, which through evaluation of the arterial wall structure and thickness allows minimisation of the drawbacks of routine angiography.
Intravascular ultrasound
In recent years, IVUS has made a growing contribution to coronary artery disease diagnosis and treatment, and its use to guide percutaneous coronary interventions (PCI) has been associated with better clinical outcomes than coronary angiography-based PCI [23]. Among the key features supporting the use of IVUS are a direct evaluation of the arterial wall, deep tissue penetrance (4–8 mm), a high axial resolution of < 40 µm in the newer-generation models, and the tomographic perspective derived from the examination. All the aforementioned benefits result in the ability to precisely quantify such parameters as plaque size, lumen area, and (partially) its composition. The allowance to measure the thickness of the intimal layer allows the identification of the development of CAV in its early stages, when its presence is not detectable in angiography. In patients after OHT, IVUS examination was initiated in the last decade of the 20th century with the study by St Goar et al., in which significant intimal thickening in the population with normal angiogram 1 year after OHT was found [24]. It has been shown that the occurrence of any-stage CAV in 1-year follow-up after OHT can reach 58% when evaluated with IVUS, in comparison with 10–20% in the coronary angiography [1, 25].
Based on the studies performed to date, clinically significant CAV should be determined once the intimal layer width exceeds 0.3 mm or when the combined width of the intimal and medial layer is greater than 0.5 mm [26]. There is evidence that rapid progression of CAV, specified as an increased maximum intimal thickness, by at least 0.5 mm 1 year after OHT is associated with an elevated risk of death and nonfatal major adverse cardiac events [27, 28]. The cause of such a bad prognosis is mostly explained through excessive immune response in the early period after OHT, which results in the most profound intimal thickening at this time.
Significant penetrance of IVUS enables accurate volumetric quantifications of the coronary plaque. In the pilot study conducted at the Cleveland Clinic the percentage of atheroma volume (PAV) increased by 3.11% in the first year after OHT, which contrasts with the 1% increase of PAV in the overall population per year [18]. According to the serial 3D volumetric IVUS performed at baseline and 1 year after OHT in the study by Okada et al., paradoxical remodelling of the arterial wall (increase in the intimal volume with a decrease in the overall volume of the vessel) was an independent risk factor of death or repeat heart transplantation [19]. Interestingly, intimal thickening was more pronounced in the proximal LAD, while vascular remodelling was observed on the entire vessel, which differs from an increase in the size of the vessel compensating luminal narrowing in native CAD.
There are certain limitations of IVUS utilisation in the assessment of CAV. First of all, a relatively high diameter of the IVUS catheter restricts its usage only to the epicardial arteries with sufficient lumen. As mentioned previously, the initial phase of CAV often occurs in the small branches of the arterial tree, which are inaccessible for an IVUS catheter. Second, the usual IVUS protocol involves assessment of a single artery, a practice which, in the past, has been associated with underestimation of CAV prevalence. As reported in the study by Kapadia et al., the examination of all three epicardial vessels resulted in a more than two-fold increase in the CAV detection rate (58% vs. 27%) 1 year after OHT [25]. Furthermore, only limited information regarding the composition of the arterial wall could be derived from the IVUS examination.
Finally, there is evidence indicating that the measurement of intimal width does not necessarily go in hand with pathological findings in coronary microvasculature, suggesting an insufficient direct correlation between these two results [29].
Virtual histology
In order to overcome the information gap on the composition of the arterial plaque in IVUS examination, virtual histology intravascular ultrasound (VH-IVUS) has been introduced. The rationale of this technique is based on the spectral analysis of radiofrequency data derived from IVUS assessment, which differentiates four major types of lesions (namely: fibrous, fibrofatty, calcified, and necrotic core). According to the studies performed in patients with native CAD, in vivo and ex vivo accuracy of VH-IVUS in the qualitative characterisation of plaque components was, respectively, 87–97% and 94–97% [30, 31].
In a study conducted on 67 patients after OHT, the histological components of the arterial wall affected by CAV were correlated with time from OHT [24]. In a longer follow-up, the proportion of fibrous and fibrofatty tissue decreased, whilst the percentage of necrotic core and calcification in the plaque was increasing, suggesting the transition into an ‘atherosclerosis-like’ image of the plaques in the long-term follow-up. A significant correlation was also found between VH-IVUS results and the presence of some clinical factors, such as diabetes or male gender, which were associated with a higher proportion of necrotic core elements in long-term follow-up [32].
Raichlin et al. classified plaques containing 30% or more of necrotic core and dense calcium as inflammatory, whilst those below the threshold of 30% were classified as non-inflammatory [33]. As stated by the authors, the presence of inflammatory plaques was associated with a significant increase in their dimensions, an accelerated progression of CAV, and, finally, a higher risk of early recurrent rejection of the transplanted heart.
There are specific limitations to the VH-IVUS approach. First, the majority of data on the utility of VH-IVUS are derived from observational data, with scarce evidence derived from prospective randomised clinical trials [34, 35]. Therefore, the quality of scientific literature confirming its value is still poor, and further studies are mandatory for its confirmation. Second, the ability of VH-IVUS to detect and identify specific elements of coronary plaque is significantly decreased in the presence of intimal hyperplasia (IH). Since the initial pathomechanism of CAV development is based on IH, it could significantly suppress its wider use in patients after OHT.
Optical coherence tomography
Optical coherence tomography is a novel approach utilising long-wavelength, near-infrared light. The clinical utility of OCT led to its introduction in multiple medical specialities, such as ophthalmology, dermatology, neurology, and gastroenterology. OCT provides unsurpassed resolution of analysed tissues, which, in the state-of-the-art devices, can be as low as < 10 µm, which is approximately five times the resolution of IVUS [36]. A comparison of IVUS and OCT is described in Table III and presented in Figure 1. Apart from improved plaque characterisation, one of the key advantages of OCT over IVUS is significantly lower interobserver variability, which after inclusion of more specified 3-D algorithms could be even further decreased [37, 38].
Figure 1
Markers of vulnerability in atherosclerotic plaque by OCT, matching IVUS of the same area, and measurement of quantitative macrophage scores by OCT. OCT images reveal vulnerable features of plaque (indicated by an asterisk), such as a lipid pool (A), thin-cap fibroatheroma (B), macrophages (C), and microchannels (white arrows) (D). Matching IVUS image of the same area of the OCT is also indicated (asterisk). In the clustered macrophage, the arc length was calculated using the mean luminal diameter and the arc angle (E). The total sum of each arc length was determined as a point of the quantitative macrophage score
IVUS – intravascular ultrasound, OCT – optical coherence tomography.
Reprinted with permission from Park et al. [50]
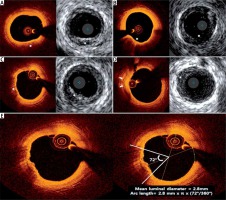
Table III
Comparison of IVUS vs. OCT
Considering the nature of cardiac allograft vasculopathy and unsurpassed precision and accuracy of OCT, it can provide an additional diagnostic tool in the early identification of CAV [39–41]. The selection of studies on CAV performed with the use of OCT is presented in Table IV. The normal thickness of intima is significantly below the resolution of IVUS, and subtle changes at the initial stages of CAV might be detectable only with OCT. Because their usual presentation is increased proliferation of the intimal layer, an intima-media ratio of > 1 has been proposed by Khandhar et al. to identify patients with an already progressed disease [40]. However, mostly due to lacking therapeutic possibilities, it is still of unknown significance whether early detection of CAV improves outcomes in patients after OHT or not.
Table IV
Selected studies of cav assessment by OCT
First author, year | Number of patients | Aim of the study | Results |
---|---|---|---|
Garrido IP, 2012 [38] | 21 ≥ 1 year after OHT | Comparison of OCT and IVUS in correlation with laboratory markers and CFR findings | No significant differences between OCT and IVUS for MIT and LA More thorough morphological identification of contents of arterial wall Lower interobserver variability of parameters in OCT |
Khandhar SJ, 2013 [40] | 15 as routine follow-up 1–4 years after OHT with no CAV in CCA | Evaluation whether subtle early intimal thickening can be detected and assessed qualitatively in OCT | In 8 (53.3%) patients despite proper angiographic image abnormally thick intimal layer was found IMR > 1 was defined as threshold of abnormality for early detection of CAV In 7 (46.7%) patients lipid-rich or calcified plaques were found |
Cassar A, 2013 [44] | 53 as routine follow-up consisting of CCA and IVUS analysis | OCT evaluation of plaques in LAD determined in IVUS | Strong correlation between OCT and IVUS for MIT No superiority of OCT vs. IVUS in CAV diagnosis rate (72% vs. 72%) OCT allowed to determine in the CAV lesions additional features typical for native atherosclerosis, vulnerable plaques and complicated lesions including intracoronary thrombi |
Dong L, 2014 [53] | 48 as routine follow-up | Correlation of OCT results with the history of cellular rejection | Significant difference in prevalence of each analysed segment attenuation (prox/mid/dist) with foamy macrophages/lipid droplets/intimal microvessels between patients with NMGR and HGR. Significantly higher mean IT in the main vessel and side branches, and lower lumen area in HG patient |
Aoki T, 2015 [45] | 8 as routine follow-up 1 year after OHT | Evaluation of VV in OCT | Strong correlation between %MCV and %PV suggesting that microchannels play significant role in development of CAV |
Ichibori Y, 2016 [46] | 45 as routine follow-up: | Evaluation of CAV with IVUS and OCT in all three coronary vessels | In OCT at 1 year after OHT, the presence of MCs was higher than at 8 weeks after OHT (39.1% vs. 10.7%, p = 0.023) with no such differences in longer follow-up The majority of MCs occurred in RCA and LAD MC occur mostly in thicker intima (> 0.5 mm) mostly in eccentric intimal plaques Diameter of MCs correlated with intimal burden Risk factors for MC development included donor age, CMV infection, diabetes, LDL-C, and width of intima |
Park KH, 2016 [47] | 19 as routine follow-up | Determination of relationship between VV density and changes in intimal CAV plaque volume | At baseline OCT, in CAV areas %VV consisted of mean 3.6 ±0.9% of the vessel volume. In IVUS, 12-months later, PV in those regions increased significantly. Changes in %PV were independently associated with time from OHT (in years) |
Shan P, 2016 [49] | 60 as routine follow-up with need for OCT: | Comparison of morphology of coronary lesions in LAD in patients with HGR and NMGR vs. patients with NCA | Patients with HGR had lower LA, EEL and IEL area than patients with NCA and NMDR Width of media and intima were significantly lower in patients with NMDR than in the rest Distribution of the plaques was significantly different between the groups: in patients with NCA worst LA were significantly lower than in patients with CAV, while CAV lesions were more homogeneous in entire CA tree Patients with HGR had higher macrophage infiltration on the entire length of LAD |
Park KH, 2017 [50] | 34 as routine follow-up | Evaluation of influence of plaque vulnerability markers on CAV progression | VS assessing lipid pools, TCFA, macrophages and microchannels was performed. Plaques with higher VS were at significantly higher risk of volume increase in 12 months, and VS was the only risk factor of volume increase in multivariate analysis |
Clemmensen TS, 2017 [51] | 62 as routine follow-up | Characterisation of CAV phenotypes in multi-vessel OCT on the progression of CAV | CAV progression prediction model was generated. The vessel factors included lipid plaque, calcification, layered fibrotic plaque, bright spots, narrowed LA, thickened intima and elevated LIR and IMR. The most prevalent components of CAV plaques were layered fibrotic plaques |
Clemmensen TS, 2018 [41] | 26 as routine follow-up at 3 months and repeatedly at 12 months after OHT | Identification of changes in arterial wall morphology in the first year after OHT | In 45% of patients, abnormalities in vascular wall structure were found at 3 months post-OHT Mean IT increases by 20% to the value of 1.9 mm in the first year after OHT, but LA decreased only by 2% in that time. Presence of layered fibrotic plaques increased almost 5 times to 4.8% with no significant difference in extent of lipid or calcified plaque components |
Pazdernik M, 2018 [37] | 50 as routine follow-up at 1 month and repeatedly 12 months after OHT | Determination of a novel method to assess IT and MT with OCT | With the use of 3D LOGISMOS graph-based approach and JEI method, boundaries of each layer in the coronary artery wall were identified with significantly higher precision than without those algorithms. Thus, quantification of the required elements with 3-dimensional imaging was possible The method allows quantification of even very subtle, location-specific changes in the arterial wall morphology over time |
[i] CA – coronary artery, CAV – cardiac allograft vasculopathy, CCA – conventional coronary angiography, CFR – coronary flow reserve, CMV – cytomegalovirus, EEL – external elastic lamina, HGR – high grade rejection, IEL – internal elastic lamina, IMR – intima-media ratio, IT – intimal thickness, IVUS – intravascular ultrasound, JEI – just enough interaction, LA – lumen area, LAD – left anterior descending artery, LIR – lumen/intima ratio, LVSD – left ventricular systolic dysfunction, MC – microchannel, MCV – microchannel volume, MIT – maximal intimal thickness, MT – medial thickness, NCA – native coronary atherosclerosis, NMGR – none/mild grade rejection, OHT – orthotopic heart transplantation, PV – plaque volume, TCFA – thin-cap fibroatheroma, VS – vulnerability score VV – vasa vasorum.
Although the study by Hou et al. was conducted on just seven long-term survivors after OHT, the OCT analysis allowed significantly more frequent detection of intimal hyperplasia in comparison with IVUS (66.7% vs. 14.3% of analysed LAD segments) [42]. This result requires attention because the minimal time from transplantation to OCT analysis was 1.5 years, which suggests that evaluation of even long-term OHT recipients with IVUS may be insufficiently sensitive to identify development and progression of CAV.
One of the unique virtues of OCT, mostly due to the significantly shorter wavelength it is emitting, is the ability to clearly differentiate the wide variety of vascular wall components and accurately depict intima-media interface. In contrast to VH-IVUS, specific contents of vascular wall can easily be identified in OCT, including lipid-rich, fibrocalcified, and fibrous tissues. The most pronounced identificational difference between OCT and any other available intravascular imaging method is the ability to assess thin-cap fibroatheromas and lipid-rich plaques, which are widely perceived as contents of high-risk, vulnerable plaque [43, 44]. In the study by Cassar et al., despite, surprisingly, no significant differences in the quantitative assessment of intima-media thickness between IVUS and OCT, the latter allowed the identification of various morphological components of CAV plaque, which before had been attributed only to native coronary atherosclerosis [44]. The authors concluded that CAV should be perceived not solely as a diffuse fibrotic disease but as its combination with native coronary atherosclerosis, including the contribution of calcifications and the presence of lipid pools. Therefore, the results are consistent with those proposed with the use of VH-IVUS, although their accuracy is significantly higher. Further nuances of CAV-affected arterial wall were identified with OCT, such as the influence of early development of vascular vasa vasorum, on the future progression of the disease [45–47]. Macrophage infiltration, which is clearly visible in OCT, is another important factor of plaque vulnerability [48]. In the study by Shan et al., patients with high-grade organ rejection had significantly higher infiltration of LAD with macrophages in comparison with participants with either no/mild rejection or patients with native atherosclerosis [49].
Taking into account the ominous outcomes in patients with CAV, the most novel studies on the use of OCT in this group determined several risk factors of CAV progression. In the study by Park et al. the presence of vulnerable plaque, consisting of lipid pools, macrophages, microchannels, and thin-cap fibroatheroma, was the sole risk factor of plaque volume increase in multivariate analysis [50]. Astonishingly, time in years after OHT, donor age, and ischaemic time, which had previously been considered as crucial risk factors for CAV progression, did not reach significance in multivariate analysis, which might suggest other, still unknown mechanisms of CAV development after OHT. In contrast, the CAV progression prediction model generated by Clemmensen et al. included both patient- and vessel-related risk factors [51]. Among the vascular factors absent in the analysis by Park et al., lumen area narrowing, intimal thickening, and elevated lumen-intima, along with intima-media ratios were identified. However, when adjusted for an angiographic grade of the disease, the association between the presence of vulnerable plaque elements, such as layered fibrotic plaque (morphological presentation of endothelial healing) or bright spots (OCT image of macrophage activation), did not reach statistical, but numerical significance (p = 0.06). Therefore, although studies utilising OCT contributed to a better understanding of the mechanism of CAV, current knowledge remains insufficient, and further studies are warranted to explore this complicated scientific phenomenon.
Limitations of optical coherence tomography
Although OCT has, to some degree, revolutionised our current perspective on CAV development and progression, it possesses significant limitations. First, even though multiple studies have been conducted to date, the clinical utility of CAV monitoring other than more accurate surveillance has not been presented by any of them. OCT analysis did not change the therapeutic approach in any of the aforementioned studies; therefore, its usefulness in routine practise remains questionable.
Second, despite the dramatic difference in image resolution between OCT and IVUS, the vascular wall penetration obtained in OCT imaging is significantly lower and often does not allow the identification of structures located deeper than 2 mm from the wall border. Adding the fact that, in the currently available literature, plaques thicker than 1.3–1.5 mm are difficult to measure, especially when their fibrous cap is thickened, OCT does not seem to be an appropriate diagnostic tool for long-term monitoring of patients with a progressed disease [52].
Furthermore, to clear the lumen of the coronary artery from blood to visualise the vascular wall, a significant volume of contrast medium is necessary, which could result in contrast-induced acute kidney injury. Patients after OHT are often exposed to multiple risk factors of renal impairment, including nephrotoxicity of immunosuppressive medication, increased age, and diabetes. According to worldwide data, the prevalence of chronic kidney disease in patients after OHT varies between 51.1% and 68.4% in 5- and 10-year follow-up. Therefore, one has to be extremely cautious not to exert contrast-induced nephropathy (CIN) due to excessive dye volume used in this susceptible group of patients. In this cohort of patients, CAV assessment with IVUS seems to be the most logical alternative, mostly because IVUS image acquisition does not require dye injections. Studies conducted to date among patients with native coronary artery disease confirmed that IVUS-guided coronary procedures can be safely performed with significantly lower use of contrast medium [53–55].
Fractional flow reserve
Although it is not an imaging modality, the physiological assessment of coronary artery disease, such as fractional flow reserve (FFR), has become one of the primary procedures to evaluate the necessity of coronary intervention [56]. In brief, it quantifies the pressure difference between the proximal and distal segments of the diseased artery in the conditions of drug-induced hyperaemia, and thus assesses the severity of the flow obstruction in the artery.
In the context of FFR, there is an important aspect of CAV pathomechanism and presentation that requires attention. Namely, its utilisation in non-CAV patients is mostly confined to focal stenoses. In the largest trials investigating the use of FFR in the assessment of CAD severity, the majority of lesions were angiographically detected focal stenoses, even if located in multiple coronary arteries [57, 58]. Therefore, as the CAV develops more diffusely – often with no angiographically determinable stenoses – and the revascularisation strategies post-OHT do not bear the optimistic results, the use of FFR in the assessment of CAV severity is also restricted.
However, in two analyses by Fearon et al., in patients who underwent FFR and IVUS assessment, even in patients without severe angiographic stenoses, there was a certain percentage of patients with an FFR ≤ 0.80 [59, 60]. FFR values correlated with the majority of IVUS parameters, with the highest coefficients observed between the FFR results and the indices of plaque burden, such as plaque area and volume [59, 60]. In patients with FFR ≤ 0.80, the average plaque volume oscillated around 40%, in comparison with around 20% in patients with an FFR > 0.80. The correlation coefficients between the FFR values and the indices of focal stenoses were much weaker [59]. However, in both studies the FFR results were relatively discordant and no clinical predictors of baseline FFR could be found [60]. Even though the authors demonstrated recently that FFR ≤ 0.90 immediately after OHT was an independent predictor of late death or retransplantation, these findings still require validation [61].
Hence, it seems that, although routinely used in non-CAV patients, the use of FFR in the detection of CAV possesses low consistency and its results are not as precise as those of intracoronary imaging, especially more than a year after OHT, when its results do not correlate with IVUS measurements [62].
Conclusions
Current guidelines of ISHLT on the management of patients after heart transplantation support the use of conventional coronary angiography as the gold standard for CAV diagnosis, mostly, due to its cost-effectiveness, wide availability, reproducibility, and low rate of complications. That is why current ISHLT criteria for identification and staging of CAV are also based on coronary angiography findings. More accurate tools such as optical coherence tomography and other intravascular imaging modalities have recently been studied, allowing the diagnosis of CAV at a significantly earlier stage of progression. The advantage of optical coherence tomography in the assessment of CAV is its unrivalled resolution and ability to differentiate various components of coronary plaque; however, because the treatment options for this condition remain limited, the clinical value of early CAV diagnosis remains uncertain. Therefore, further studies are required to confirm the clinical value of detection of CAV by OCT at its earliest stage in reducing the risk of clinical endpoint occurrence in the population after OHT.