Photoplethysmography
Photoplethysmography (PPG) measures volumetric changes in blood in peripheral microcirculation. PPG is simple, painless and inexpensive and it does not require much experience of the person who conducts the test [1]. PPG allows only for a point assessment of microcirculation and the result of the test is not given in absolute units. PPG involves a probe equipped with a source of infrared light and a set of optic sensors. The infrared light is absorbed by the examined tissue. Depending on the volume of blood at the moment of the test in the examined skin fragment, more or less light is absorbed. In consequence, the amount of the light reflected relates to the point changes in the blood volume. Thus, the alterations in blood flow can be registered as alterations in light intensity [2]. The measurement may be performed on fingers and toes on both hands and feet. The test is conducted in a silent room at > 20ºC temperature, allowing time for the end of the vascular response, connected to the locomotive muscle tension and heat adaptation.
The PPG shows alterations in blood flow in the form of a chart presenting point alterations in the volume of the flowing blood. Its waveform consists of two phases: the rapid anacrotic phase and the slow catacrotic phase (Figures 1, 2). The anacrotic phase is primarily related to systole, while the catacrotic phase is related to diastole and reflexes from the periphery. Typically in the subjects with healthy artery compliance we see a dicrotic notch in the catacrotic phase [3]. Reduction in microcirculation flow detected in the course of the photoplethysmography exam can show growth retardation, rounded top and a very slow decline without the dicrotic notch [4].
Photoplethysmography is currently the most commonly used method of diagnosis of chronic venous insufficiency. It allows for the assessment of the functioning of valves in the superficial and deep vein systems by the measurement of the venous refilling time (VRT). The photoplethysmographic test can also be applied in diagnostics of peripheral arterial occlusive disease. Allen et al. have demonstrated the clinical value of photoplethysmography pulse measurements on the toes of both feet in the diagnostics of the disease in the lower limbs [5]. PPG may be also used in the diagnostics of alopecia areata (AA) in order to confirm the vascular aetiology of this disease. Sudnik has demonstrated alterations in the photoplethysmographic curve denoting mild flow distortions in 63.5% and severe flow distortions in 36.5% of AA patients [2].
Orthogonal polarization spectroscopy
Orthogonal polarization spectroscopy (OPS) is a relatively new technique of human microcirculation imaging without using fluorescent dyes. OPS imaging shows a wide range of clinical applications with a strong emphasis on establishing the precise diagnosis, prognosis and treatment implementation [6]. Polarized light with wavelength of 548 nm, which is reflected from the examined tissue, is captured by a camera and used to obtain the optimal microcirculation imaging in OPS. The light scattered by the superficial layer of tissue is blocked in the process of second polarization. As a consequence, only the light returning from the deeper layers of tissue passes second polarization and improves visibility of erythrocytes. Computer analysis of erythrocyte movement enables assessment of perfusion, diameter of vessels and capillary density [6].
The orthogonal polarization spectroscopy technique can be applied not only for real-time studying of microcirculation in skin vessels, but also for submucosal microcirculation. The test is usually conducted in the sublingual area and skin of newborns. Moreover, OPS technology allows for examination of tissues covered by a thin epithelial layer and internal organs during surgical operation [6]. The obtained measurements have rather a quantitative character, however, OPS results are susceptible to artefacts due to organ movement and changes in blood pressure. De Backer et al. using the OPS technique have proved significant changes in skin microcirculation in patients with sepsis and septic shock [7]. OPS proved to give a more objective visualization of microcirculation in critically ill patients compared to videocapillaroscopy and laser Doppler techniques [8]. Orthogonal polarization spectroscopy has been also applied in monitoring of hypovolemic patients and in term and preterm infants as an effective non-invasive method of tissue perfusion assessment. Further development of the OPS is bound to bring a broader clinical application of this method, especially in the field of surgery, mainly neurosurgery and plastic surgery, as well as neonatology, anaesthesiology and dermatology.
Near-infrared spectroscopy
Near-infrared spectroscopy (NIRS) is a specific form of tissue reflectance spectrophotometry. This method uses infrared light of 700–1000 nm which easily penetrates soft tissues and is subsequently partly diffused and partly absorbed by chromophores such as haemoglobin, myoglobin and cytochrome aa3. On this basis the tissue haemoglobin oxygen saturation (tHbO2) assessment is performed [9].
This technique may be used for assessment of various organs, mostly for the measurement of blood flow through the brain and muscle tissue. Advantages of this method are the simplicity of use and short time of examination. The devices for clinical measurement which are currently available are easy to operate. Unfortunately, NIRS does not allow for measurements of absolute values of tHbO2. In addition, the resolution of the signal is poor and the exact depth of light tissue penetration is not known [9]. Originally this method was used for continuous monitoring of tissue haemoglobin oxygen saturation in the brain during carotid endarterectomy [9].
NIRS technique is also currently recognized as a good tool for assessing superficial tissues, including skin microcirculation. NIRS is used primarily in critically ill patients for monitoring oxygen supply to the skeletal muscles in ICUs. Lima and Bakker proved that monitoring of circuit perfusion may show early indications of tissue hypoperfusion [10]. The NIRS method has been reported to assess skin microcirculation in patients with sepsis and in septic shock, however its prognostic value has not been confirmed yet [11]. Hartwig et al. in a pilot research successfully used this technique for the assessment of microcirculatory dysfunction in systemic sclerosis both in static and dynamic conditions. They also demonstrated clinical use of NIRS in assessment of skin microcirculation in connection with vascular occlusion testing [12]. NIRS, similarly to laser Doppler flowmetry and transcutaneous oxygen tension, may be applied in the assessment of microcirculation in patients with diabetic foot [13]. The assessment of microvasculature may help to make a therapeutic decision [14].
Tissue reflectance spectrophotometry
Tissue reflectance spectrophotometry (TRS) is based on the detection of backscattered light in specific wavelength spectra for oxygenated haemoglobin at two peaks of 542 nm and 577 nm and deoxygenated haemoglobin at a peak of 556 nm. This technique allows for the assessment of haemoglobin oxygen saturation and haemoglobin concentration in capillaries so that it reflects skin microcirculation functioning in real time [9]. Measurements may be taken from the surface of any organ, although for obvious reasons it is most commonly performed on the surface of the skin and gastric mucosa. A short time of examination, expression of the results in absolute units and the possibility to perform repetitive measurements are the main advantages of TRS. Limitations include the fact that the result depends on presence of tissue chromophores other than haemoglobin i.e. melanin or cytochromes [9]. Erroneous results in measuring blood content and oxygenation might occur in persons of significant skin pigmentation as melanin in the epidermis reduces the amount of backscattered light and has a characteristic absorption spectrum, different in every person [15]. Thus, TRS is presently applied predominantly for assessment of tissue healing.
Optical coherence tomography
Optical coherence tomography (OCT) is a developing technique of imaging, mainly used in medical diagnostics. This method allows for non-invasive penetration into the examined tissue, which is why it is also called optical biopsy [16]. Optical coherence tomography uses the phenomenon of interferometry of light with the tissues. In the OCT method, sources of light of special coherence and spectrum of considerable spectral width. Light used in this method has an intensity lesser than a couple of milliwatts. Thus, the OCT examination is entirely non-invasive and it may be safely applied repeatedly in many places. This technique is analogous to the ultrasonographic technique, with the use of light instead of sound [17]. The most important advantage of this method is high resolution of 10–20 μm, allowing for obtaining images comparable to histopathology. Other advantages include the simplicity of use of the device, imaging in real time and capability of continuous registration. The OCT does not require prior preparation of a patient. Unfortunately, OCT is most effective for optically transparent tissue assessment. It covers a relatively small surface and is limited to imaging only 1–2 mm into the surface of the tissue (Figure 3).
Figure 3
The optical coherence tomography scan of normal retina (with the permission of L. Glasner MD, Medical University of Gdansk, Department of Ophthalmology)
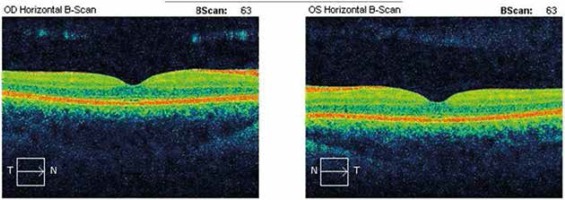
The OCT is a constantly developing method and is applicable in many fields of medicine. In ophthalmology it is regarded as the most modern technique of imaging in diseases of retina and anterior segment of the eye. In patients with diabetic retinopathy, OCT is used as an objective monitoring technique of the macular thickening before and after therapy as well as for vitreous assessment [18, 19]. In interventional cardiology it is applied in the coronary artery disease diagnostics. In dermatology, OCT is used to assess the extent of tissue damage, to measure pathologic skin lesions such as angioma and for precise assessment of scars and wrinkles. Comparison of correlation mapping optical coherence tomography (cmOCT) performed on the forearm of healthy persons and psoriasis patients demonstrates characteristic microcirculatory alterations in the presence of psoriasis (Table 1).
Table 1
Non-invasive methods of assessment of microcirculation