Sarcopenia is associated with high mortality and a high complication rate. Given the aging in the Western population, sarcopenia represents a high health care system and socio-economic burden and affects the quality of life. The prevalence of sarcopenia depends on the used definition of sarcopenia, but figures up to 33% for individuals living in long-stay care facilities and up to 29% for community-dwelling elderly persons have been reported [1, 2].
Abdominopelvic CT is performed in various clinical indications. Availability of abdominal and pelvic CT imaging allows assessment of the psoas area (PMA) and skeletal muscle area (SMA) to assess muscle mass without additional radiation load. Further, the muscle quality can be estimated by measuring the CT attenuation coefficient of the psoas or skeletal muscle (Figures 1 and 2).
FIGURE 1
Panel A shows an example of a patient suffering from sarcopenia. Panel B shows an image of a non-sarcopenic patient. Note the difference in psoas muscle volume. Note the difference in psoas muscle cross-sectional area (white arrow) between the two patients
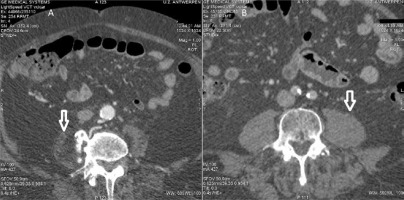
FIGURE 2
Measurements of the psoas muscle area (PMA) and muscle quality. Panel A shows the manual contouring of the region of interest (ROI), being the right PMA. Panel B: Within the ROI, a filter selects all voxels with a CT attenuation coefficient within a predefined range corresponding to skeletal muscle. Similarly, the filter can also select all voxels within the ROI with a CT attenuation value attributed to the low-density muscle (LDM). In this case, LDM was defined as the PMA portion with a CT-attenuation coefficient ranging from 0 to 29 Hounsfield units
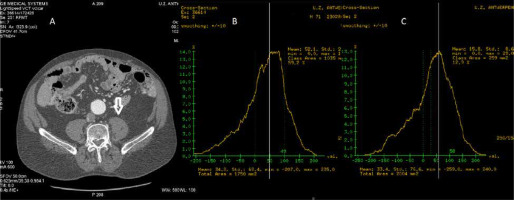
This paper discusses the definition of sarcopenia by the European Working Group on Sarcopenia in Older People (EWGSOP). Section 2 briefly discusses assessing sarcopenia by magnetic resonance imaging, ultrasound, and bioelectrical impe-dance analysis. The advantages and disadvantages of these diagnostic modalities are explained.
The definitions of CT-measured psoas muscle index (PMI), skeletal muscle index (SMI), and transverse psoas muscle thickness (TPMT) are discussed in Section 3.
Finally, we review the literature studies on PMI and SMI in different clinical settings. The studies are grouped by cardiovascular settings, vascular disease, trauma, and ICU/emergency medicine.
SECTION 1. DEFINITION OF SARCOPENIA
In 2010, sarcopenia was defined by the European Working Group on Sarcopenia in Older People EWGSOP) as “a syndrome characterised by progressive and generalised loss of skeletal muscle mass and strength with a risk of adverse outcomes such as physical disability, poor quality of life, and death” [3].
In a 2019 EWGSOP2 consensus document, the progress and new insights in the field of sarcopenia were summarised in 4 key-points [4]:
For a long time, sarcopenia has been associated with aging. Nowadays, it is recognised that sarcopenia begins to develop earlier in life [5]. Due to evolving insight, the “sarcopenia phenotype” is supposed to have several contributing aetiologies beyond ageing [6], allowing the development of new treatment strategies to delay the progression of sarcopenia.
According to the current insights, sarcopenia is a muscle disease. In the pathophysiological thinking about sarcopenia, low skeletal muscle mass as the principal determinant has shifted towards low muscle strength [7–9]. It was emphasised that this change might facilitate the detection of sarcopenia in clinical practice, as muscle strength can be assessed without sophisticated diagnostic tools.
It has been recognised that sarcopenia is asso-ciated with low muscle quantity and quality. However, these parameters of muscle quality are mainly used in the research setting rather than in clinical practice [10–12].
Sarcopenia is underdiagnosed and undertreated. There is a lack of consensus on which variables should be measured, how they should be measured, and which thresholds should be used to diagnose sarcopenia. Further, it is unclear how therapeutic interventions should ideally be evaluated [13].
Based on these new lines of evidence, the EWGSOP introduced a new operational 3-tier definition of sarcopenia in 2018. This definition recognises muscle strength as the primary factor in sarcopenia. The 3-tier definition of sarcopenia identifies the presence of 3 criteria below [4]:
The diagnosis of “probable sarcopenia” is made if the patient fulfils Criterion 1. Diagnosis of sarcopenia is confirmed if additional workup confirms Criterion 2. If all three criteria are met, sarcopenia is scored as severe [4].
SECTION 2. ASSESSMENT OF SARCOPENIA
This paper focuses on the anthropomorphic assessment of the skeletal muscles using abdominopelvic computed tomography (CT) imaging, which will be discussed in Sections III and IV. This section will discuss other diagnostic modalities and fundamental principles necessary for a good understanding of the sarcopenia literature but does not belong to the core of this article. Therefore, this section does not aim for an in-depth review or completeness.
Assessment of muscle strength and performance tests and SarQol questionnaire
The EWGSOP recommends two measurements to assess muscle strength: grip strength and the chair stand test (Table 1).
TABLE 1
Clinical tests and questionnaires recommended by the EWGSOP for the assessment of sarcopenia
I. Assessment of muscle strength The EWGSOP recommends two measurements to assess muscle strength: grip strength and the chair stand test. |
1. Grip strength is recommended for several reasons: – Low grip strength is a strong predictor of adverse outcomes such as mortality, poor health-related quality of life, increased functional limitations, and extended hospital stays [8]. – Measuring grip strength is cheap and straightforward, thus suitable for routine use in hospital practice, as well as in community healthcare [8, 86–88]. – Grip strength is measured using a calibrated handheld dynamometer under strictly defined test conditions. Normal reference values derived from appropriate reference populations have been provided [89]. |
2. The chair stand test – The chair stand test is used as a proxy for the strength of the quadriceps muscle group. – The chair stand test measures a patient’s time to rise five times from a seated position. The patient is not allowed to use the arms during the test [4]. – The chair stand test performance requires both strength and endurance from the patient. |
II. Physical performance The EWGSOP defines physical performance as “an objectively measured whole-body function related to locomotion”. This multidimensional concept involves not only the skeletal muscles but also central and peripheral nerve function [4, 14]. The EWGSOP 2019 consensus paper propagates four tests to quantify physical performance: gait speed, the 400-m walk test, the Timed-Up and Go test (TUG), and the Short Physical Performance Battery (SPPB) [4]: |
1. Gait speed – Gait speed is considered a highly reliable, quick, and safe test for sarcopenia [88]. – In patients with sarcopenia, gait speed is a predictor of adverse outcomes such as sarcopenia-induced disability, need for institutionalization, cognitive impairment, falls, and mortality [90]. – The 4-m usual walking speed test is usually used to measure the gait speed. The EWGSOP2 recommends a single cut-off speed of ≤ 0.8 m s–1 to indicate severe sarcopenia [4]. |
2. The SPPB – The SPPB is a composite test combining gait speed measurements, a balance test, and a chair stand test [88]. A maximum score of 12 points can be obtained, with a score of ≤ 8 points indicative of poor physical performance [3, 88]. |
3. Timed-Up and Go test (TUG) The TUG test requires that the tested individuals rise from a standard chair, then walk to a marker 3 m away, turn around, and walk back to sit down again [91]. |
4. The 400-m walk test The 400-m walk test evaluates walking ability and endurance. The participants must complete 20 laps of 20 m, each lap as fast as possible. Two rest stops are allowed during the test [4]. |
These four physical performance tests can be performed in most clinical settings. For practicality, the EWGSOP2 recommends the gait speed to evaluate the physical performance to predict sarcopenia-related outcomes [92]. |
III. Quality of life questionnaire |
SarQoL questionnaire The SarQoL tool is a questionnaire for patients with sarcopenia and helps physicians assess the patient’s perception regarding his or her physical, social, and psychological health [15, 16]. SarQoL identifies and predicts sarcopenia complications that may impact the quality of life in the later stages of life. The SarQoL questionnaire has been validated as a consistent and reliable tool [15]. |
The EWGSOP defines “physical performance” as “an objectively measured whole-body function related to locomotion”. This multidimensional concept involves not only the skeletal muscles but also central and peripheral nerve function [4, 14]. The EWGSOP 2019 consensus paper propagates four tests to quantify physical performance: gait speed, the 400-m walk test, the Timed-Up and Go test (TUG), and the Short Physical Performance Battery (SPPB) [4] (Table 1).
The SarQoL tool is a questionnaire for patients with sarcopenia and helps physicians assess the patient’s perception regarding his or her physical, social, and psychological health [15, 16].
Table 1 lists and explains these muscle strength tests, performance tests, and the SarQoL questionnaire.
Assessment of muscular mass
1. Dual-energy X-ray absorptiometry (DXA)
Due to differences in tissue attenuation of X-ray photons, DXA enables quantification of the body compartments:
Lean mass not only represents skeletal muscle but also includes other tissue components such as connective tissues, tendons, and skin. DXA cannot differentiate muscle from water [17]. Therefore, DXA overestimates the muscular mass conditions with fluid retention, such as decompensated heart failure and liver cirrhosis [18].
To mitigate the effect of fluid retention on lean body mass, both appendicular (arms and legs) and upper limb lean masses have been proposed as a tool to assess muscle mass in patients with ascites. This approach (appendicular skeletal muscle measurement) excludes the abdominal compartment containing ascites and may therefore be helpful in patients with cirrhosis [19].
Low lean mass and appendicular limb lean mass (ASM), assessed by DXA, predict muscle weakness and reduced mobility but are not associated with mortality [20]. Muscle mass needs to be indexed to body habitus. Muscle mass (ASM or skeletal muscle mass (SMM)) needs to be adjusted by height [2], weight, or body mass index (BMI) [17].
The appendicular skeletal mass index (ASMI), adjusted by height, is calculated as follows [17]:
2. Bioelectrical impedance analysis (BIA)
BIA does not measure ASM directly but estimates muscle mass based on whole-body electrical conductivity. The skeletal mass is determined using a conversion equation, calibrated with a population-specific reference of DXA-measured lean mass [21, 22].
BIA equipment is widely available, portable, and affordable. Authors have stated that more validation studies regarding the prediction equations for specific populations are required, and DXA remains the preferable tool over BIA [22, 23].
Like DXA, the patient’s hydration status influences the BIA measurements.
3. Magnetic resonance imaging (MRI)
MRI can evaluate muscle quality and quantity. MRI allows separation and accurate quantification of the muscle compartments and fat distribution. Detailed anatomical muscular changes can be depicted, including fatty degeneration, oedema, and muscular atrophy [17].
MRI can be performed without administration of contrast media due to its high soft-tissue contrast and multiparametric characteristics [17].
MR spectroscopy (MRS) can assess the chemical composition of the muscles [24]. DIXON-based MRI enables selective reconstruction of water signal-only and fat signal-only images [25].
4. Ultrasound assessment of muscle
Recently, ultrasound has been introduced in clinical practice to support diagnosing sarcopenia in older adults. The EuGMS sarcopenia group approved a consensus protocol for muscle assessment by ultrasound in 2018 [26].
It has been reported that non-contractile myosteatotic tissues present as hyperechogenic structures [27]. Thus, ultrasound allows the assessment of muscle quantity and quality.
A systematic review concluded that ultrasound is reliable for muscle size assessment in older patients, including those with comorbidity [28]. Furthermore, it has been demonstrated that the validity of ultrasound-assessed muscle mass is good when compared to DXA, CT, and MRI [4].
SECTION 3. ANTHROPOMORPHIC ASSESSMENT BY COMPUTED TOMOGRAPHY (CT) MEASURED PSOAS MUSCLE AREA (PMA), SKELETAL MUSCLE AREA (SMA), AND MUSCLE QUALITY
A. Measurement of PMA, SMA, and muscle quality
Assessment of PMA, SMA, and muscle quality can be performed on abdominopelvic CT studies and does not require additional radiation exposure or supplemental iodine contrast administration. Measuring these variables only requires post-proces-sing the axial slice of interest using dedicated post-processing software.
The EWGSOP recommends assessing PMA, SMA, and muscle quality at the third lumbar vertebra level [4]. It has been shown that total body skeletal muscle mass correlates well with SMA, measured at the third lumbar vertebra level [29].
A step-way approach to determine CT-acquired PMI.
Step 1: Select the axial CT slice at the third lumbar vertebra level [4].
Step 2: Determine the region of interest (ROI) by manually contouring the PMA or SMA [30] (Figure 2 Panel A).
Step 3: A filter selects the voxels with CT attenuation coefficients attributed to skeletal muscle within the ROI. A filter setting with a threshold range of – 29 to 150 HU is commonly used to define the muscle [31]. However, many researchers have used other filter settings (e.g., the muscle was defined as vo-xels with CT attenuation coefficients ranging from 0 Hounsfield units (HU) to 100 HU, within the ROI) [30] (Figure 2 Panel B).
Step 4: The CT attenuation coefficients of all voxels attributed to skeletal muscle (Step 3) are averaged. This variable is usually called HUAC (Hounsfield Units Attenuation Coefficient), PMHU (psoas muscle Hounsfield units), and psoas muscle density (PMD) in the medical literature.
Step 5: Fatty infiltration due to muscle degeneration will reduce the HUAC, as adipocyte tissue has a CT attenuation coefficient ranging from –190 to –30 HU. Thus, lower psoas muscle HUAC represents lower skeletal muscle quality. Alternatively, muscle quality can be assessed by dividing the PMA or SMA into low-density muscle (LDM) and high-density muscle (HDM) by selecting the voxels, with a predefined CT attenuation coefficient range for LDM and HDM, within the ROI (Step 2) (Figure 2 Panel C) [30]. The different approaches of muscle quality quantification are discussed in the section below.
B. Definitions of skeletal muscle mass
There is heterogeneity in the methods and definitions used to assess muscle volume from axial CT slices (Figure 3). In a review of the radiological definitions of CT-measured sarcopenia in chronic liver disease, the most commonly used descriptions were listed as follows [17]:
FIGURE 3
The different definitions used to assess sarcopenia. Panel A and B show the contouring of the region of interest to determine the psoas muscle area (PMA) (green colour) and the skeletal mass area (SMI) (red colour), respectively. Panel C demonstrates the measurement of the transverse psoas muscle thickness (TPMT) (white line)
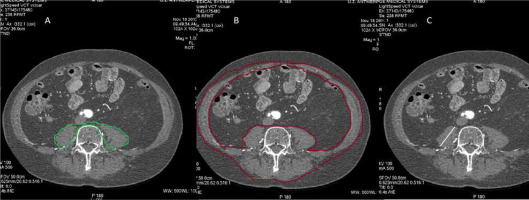
Skeletal muscle index (SMI):
Transverse psoas muscle thickness (TPMT):
Psoas muscle index (PMI):
Different indexing methods are used to adjust the SMA and PMA to the body habitus. A frequently used indexing method in research is adjusting to squared height (h2). However, there is controversy regarding the ideal indexing method for body habitus. The EWGSOP explicitly states in its consent document that it does not recommend whether PMA and SMI should be adjusted to height2, weight, or BMI [4].
Besides the issues mentioned above, comparison between sarcopenia studies measuring CT-assessed skeletal muscle quantity is often complex for other reasons. Table 2 summarises the problems encountered comparing CT-acquired anthropomorphic data.
TABLE 2
Difficulties comparing studies assessing sarcopenia by CT-measured skeletal muscle quantity and quality
1. Heterogeneity in the methods and definitions used to assess muscle volume from axial CT slices – Skeletal muscle index (SMI), obtained by indexing total skeletal muscle area (SMA) to body habitus. – Psoas muscle index (PMI) was calculated after adjusting psoas muscle area (PMA) to body habitus (usually to height2). – Transverse psoas muscle thickness (TPMT). – Occasionally, the muscular erector spinae surface area has been measured. |
2. Different indexing methods – Comparison between studies is further complicated by different indexing methods used to adjust the SMA and PMA to body habitus. A frequently used indexing method in research is adjusting to squared height (h2). – However, there is controversy regarding the ideal indexing method for body habitus. The EWGSOP explicitly states in its consent document that it does not recommend whether PMA and SMI should be adjusted to height2, weight, or BMI [4]. |
3. Selection of the axial CT slice for quantification of sarcopenia – There is no agreement regarding the lumbar vertebral level at which SMA and PMA were measured among different clinical studies. In the medical literature, studies have measured PMA at the lumbar vertebra L3, L4, or L5 levels. – It has been reported that PMA and SMA at the L3 level strongly correlate with global skeletal muscle volume [29]. The current EWGSOP 2019 consent document recommends assessing PMI and SMI at the third lumbar vertebra level [4]. |
4. Lack of use of study population-independent definitions and thresholds for sarcopenia – Authors have emphasised that many studies searching for associations between PMA and clinical outcomes have divided the study populations into PMA tertiles or quartiles rather than using study population-independent definitions and thresholds for sarcopenia [31]. – Similarly, other studies have used the median HUAC of the study population as the threshold to divide the study population into patients with high-density or low-density muscle mass. |
5. Influence of gender and ethnicity – Gender is a strong independent predictor of PMA, necessitating sex-adjusted reference values or sex-adjusted PMA subgroups in studies searching for associations between PMA clinical outcomes. – Thresholds for sarcopenia, derived from Western populations, cannot be extrapolated to an Asian population and vice versa due to differences in body constitution and lifestyle [34, 37]. |
6. Different CT attenuation filter settings have been used to define skeletal muscle – Different CT attenuation filter settings have been used to define muscle (e.g., 29 to 150 HU is commonly used to define the muscle. However, other researchers have used other filter settings ranging from 0 to 100 HU). |
Muscle quality. Besides quantification of the PMA, the psoas muscle CT-attenuation coefficient can also be measured, allowing qualitative skeletal muscle assessment. In myosteatosis, fatty infiltration will reduce the average psoas muscle attenuation coefficient, as adipocyte tissue has a CT attenuation coefficient ranging from –190 to –30 Hounsfield units (HU). In part 3A, we described how CT attenuation filters select the voxels representing skeletal muscle by setting the filter range from 29 HU to 150 HU [31] or from 0 HU 0 to 100 HU [30], depending on the definition of muscle. Similarly, the psoas muscle can be divided into low-density muscle (LDM) by selecting the muscle voxel with low CT attenuation coefficient (e.g., CT attenuation coefficients ranging from 0 to 29 HU, and high-density muscle (HDM), by using a filter setting, selecting the voxel with a CT attenuation coefficient ranging from 30 HU to 100 HU) [30] (Figure 2, Panel C).
In summary, concepts encountered in the medical literature to describe CT-acquired muscle quality are:
1. PMD (psoas muscle density): the CT-attenuation coefficient of the psoas muscle, obtained by aver-aging the CT-attenuation coefficients of the voxels of the psoas muscle; also called muscle radiation attenuation (MRA), average HUAC (average Houns-field units attenuation coefficient) or PMHU (psoas Hounsfield units) by others.
2. LDM (low-density muscle): defined as the PM portion with a low CT attenuation coefficient; other researchers used the median HUAC to divide the study population into LDM and HDM (high-density muscle); other studies defined patients in the lowest HUAC tertile or quartile of the study population as patients with poor muscle quality).
Reference values for sarcopenia using skeletal muscle CT attenuation
Reference values. The European Working Group on Sarcopenia in Older People (EWGSOP) has established thresholds for sarcopenia, obtained by dual-energy X-ray absorptiometry (DXA): appendicular skeletal mass index (ASMI) 7.0 kg m–2 and 5.5 kg m–2, for males and females, respectively [4]. The EWGSOP considers CT and MRI-measured SMI and PMA as the gold standard for muscle mass quantification, but meanwhile states that these imaging modalities should be recommended for research purposes rather than daily clinical practice [4]. The EWGSOP refers to the studies by van der Werf et al. [32] and Derstine et al. [33] for reference values of PMI and SMI (Table 3).
TABLE 3
Reference values for sarcopenia set by different organizations
DXA – dual-energy X-ray absorptiometry (DXA), ASMI – appendicular skeletal mass index, SMI – skeletal muscle index, PMI – psoas muscle index
* The EWGSOP refers to both those publications in its consensus document [4].
Patients with hepatic disease frequently undergo serial CT examinations. Given the availability of CT imaging in this clinical condition, the utility of PMI and SMI to predict outcomes in hepatic disease has been extensively studied. The Japanese Society of Hepatology [34] and the North American Working Group on Sarcopenia in Liver Transplantation [35] have established their cut-off values of sarcopenia for PMI or SMI. Thresholds for sarcopenia in the Asian population have been proposed [36]. Table 3 lists these reference values.
Gender-specific reference values. Gender is a strong independent predictor of PMI, requiring sex-adjusted PMI reference values.
Influence of ethnicity. It has been emphasised that the cut-off values for sarcopenia from Western studies cannot be extrapolated to Asian populations due to differences in lifestyle, body size, and ethnicity [34, 37].
Aetiology of sarcopenia. Some reviewers suggested that studies to define sarcopenia in hepatic disease should also be conducted according to the aetiology of the disease [17].
Technical aspects influencing sarcopenia assessment by CT psoas muscle attenuation and correlation with other tools to diagnose sarcopenia
Technical aspects of the CT-scanning protocol, such as tube current, slice thickness, and dye administration, influence the CT-measured PMA [17, 38, 39]. Table 4A summarises and explains the impact of these technical considerations on the PMA measurements. Table 4B summarises the critical points about the correlation between CT-acquired PMI and other diagnostic modalities for sarcopenia.
TABLE 4
A) Technical aspects influencing sarcopenia assessment by CT psoas muscle attenuation. B) Correlation between CT muscle assessment and other diagnostic modalities to diagnose sarcopenia
A. Technical aspects influencing sarcopenia assessment by CT psoas muscle attenuation |
---|
1. Reproducibility of PMA measurements. The semi-automated measurements of PMA have excellent inter-reader and intra-reader agreement [93]. |
2. Tube current. Tube current influences the X-rays’ energy. Lower tube current will result in lower energy of the X-rays with higher attenuation of the body tissues. Tube current reduction from 140 kV to 80 kV will result in a 5.2% reduction in total skeletal muscle index (SMI) [38]. Given the ALARA (as low as reasonably achievable) principle in the state of the medical imaging to reduce the radiation load of the patients, most medical centres will not use scanning protocols with a fixed tube current. |
3. Slice thickness. PMA is influenced by slice thickness. Compared to a slice thickness of 10 mm, PMA assessment on a 2 mm slice thickness will reduce the SMI by 1.9% [39]. |
4. Contrast administration. Differences in iodine contrast administration will result in different HUAC of the muscle [17]. |
B. Correlation between CT muscle assessment and other diagnostic modalities to diagnose sarcopenia |
5. Correlation between CT muscle assessment and physical assessment. Radiological assessment does not necessarily reflect physical performance or muscular strength. In end-stage liver disease, handgrip was a stronger predictor of mortality than CT-assessed sarcopenia [4]. |
6. Comparison between CT and magnetic resonance imaging (MRI). An excellent agreement between CT and MRI measurements of skeletal muscle mass at the level of the L3 vertebra has been demonstrated [93]. It has been advocated that the measurements of skeletal muscle mass on CT and MRI are interchangeable. |
7. Correlation between CT-measured PMI and assessment by BIA. CT measured PMI is in accordance with BIAFFMI (fat-free mass index) (ICC 0.73) and BIAASMI (appendicular skeletal muscle index) (ICC 0.69), but not with mid-upper arm muscle circumference (MAMC) (ICC 0.37) [94]. Ito et al. found a positive correlation between CT-assessed PMI and BIA-measured SMI [95]. Ashton et al. demonstrated a significant positive correlation between CT-measured PMA and BIA-derived fat-free mass in a paediatric population [96]. |
Deep machine learning
Artificial intelligence (AI)-based software to determine PMI and PMD has been developed. An AI-based analysis demonstrated that skeletal muscle density at L3 was associated with progressive aortic enlargement in patients with Marfan disease [40].
Wang et al. demonstrated that deep learning techniques enable automated quantification of PMI on CT images in patients with liver cirrhosis. Furthermore, these automated PMI measurements were predictive of mortality [41].
Van Erck et al. demonstrated that fully automatic assessment of PMA at the L3 level in CT images is feasible and stated that “it is a reliable tool that offers great opportunities for analysis in large scale studies and in clinical applications” [42].
SECTION 4. PMA ASSESSMENT IN CLINICAL RESEARCH
This section summarises the findings of studies searching for correlations between PMI/SMI and outcomes after medical procedures. In Medline, the search term “psoas muscle and outcome” showed 931 hits in October 2021. Approximately 300 studies generating outcome data in different areas of medicine were found. The results were clustered according to the underlying disease or circumstance in which the CT studies were performed: cardiac disease, peripheral vascular disease, trauma, patients in the Emergency Department or ICU, patients undergoing major non-cardiovascular non-oncological surgery, post-transplantation patients, patients undergoing oncological surgery, and patients with internal diseases, as well studies performed in a paediatric population.
A. PMA assessment in patients with cardiac disease
The medical literature on studies using PMA to assess the outcomes of patients with cardiovascular disease consists of 31 studies and can arbitrarily be divided into patients undergoing transcatheter aortic valve replacement (TAVR) (17 studies) and patients not undergoing TAVR (14 studies). Table 5 lists these studies, with the main results and the number of patients included.
TABLE 5
Psoas muscle area and/or attenuation variables and outcome after cardiac intervention
A. Post TAVR | |||
---|---|---|---|
Year & Reference | Number of patients | Study results | Association (Y/N) |
2016, Mamane et al. [97] | 208 patients undergoing TAVR | – PMA was lower in non-survivors compared with survivors among women (P = 0.047) but not men (P = 0.50). – PMA was a predictor of all-cause mortality in women after adjustment for Society of Thoracic Surgeons risk (HR 0.88 per cm2; 95% CI: 0.78–0.99). | Yes |
2016, Paknikar et al. [98] | 295 patients undergoing TAVR | – Preoperative STS score (OR 91.1; P = 0.02) and PMA (OR 0.5; P = 0.024) were associated with adverse outcome. – 2-year survival was 85.7% and 93.8% in patients with and without sarcopenia, respectively (P = 0.02). – MPA was an independent predictor of late survival (HR 0.47; P = 0.02). | Yes |
2017, Garg et al. [99] | 152 patients undergoing TAVR | – PMA indexed to BSA (OR 3.19, 95% CI: 1.30–7.83; P = 0.012) and age (OR 1.92, 95% CI: 1.87–1.98; P = 0.012) predicted early poor outcome. STS score predicted 1-year mortality (HR 3.07, 95% CI: 1.93–6.23; P = 0.011). – High-resource utilization was observed more frequently in patients with PMA less than the median (73% vs. 51%, OR 2.65; P = 0.006). | Yes |
2018, Foldyna et al. [100] | 403 patients undergoing TAVR | Patients with the lowest PMA exhibited the highest hazard of mortality. | Yes |
2018, Kleczynski et al. [101] | 153 patients undergoing TAVR | In the receiver operating characteristic analysis, PMA and psoas muscle volume (PSV), were good predictors of 12-month all-cause mortality after TAVI. | Yes |
2018, Bibas et al. [102] | 82 patients after heart transplant with abdominal CT scan available | – At baseline, mean PMA was 25.7 cm in men and 16.0 in women and decreased by 8% from the first to the last available CT scan. – Adjusting for age, sex, BMI, and cardiomyopathy aetiology, every 1-cm increase in PMA was found to be associated with a 9% reduction in long-term mortality (HR 0.91; P = 0.031) and a 17% reduction in in-hospital mortality or major morbidity (OR 0.83; P = 0.014). When PMA < the sex-specific median, the risk of mortality or major morbidity increased fourfold (OR 4.29; P = 0.026). | Yes |
2018, Hebeler et al. [46] | 470 patients undergoing TAVR | – The STS predicted risk of mortality alone was poorly predictive of 1-year mortality (AUC 0.52). – Albumin was significantly associated with increased risk for 1-year mortality (P = 0.03). PMA was not significantly associated with increased risk for 1-year mortality. | No |
2019, van Mourik et al. [31] | 583 patients undergoing TAVR | Low iPMA was associated with cardiac and all-cause mortality in females. 1-year adjusted cardiac mortality HR in females for mid-iPMA and high-iPMA were 0.14 and 0.40 respectively. Similar effects were observed for 30-day and 2-year cardiac and all-cause mortality. In females, adding iPMA to surgical risk scores improved the predictive value for 1-year mortality. | Yes |
2019, Koffler et al. [44] | 1076 patients undergoing TAVR | PMAi at the level of L4 (OR 0.049, P = 0.013) was independently associated with 30-day mortality. During a median follow-up of 435 days 27.1% of patients died. PMAi of L4 (HR 0.083, P < 0.001) was independently associated with mortality during follow-up. The addition of PMAi to the STS score led to a net reclassification improvement for 30-day and cumulative mortality. | Yes |
2019, Krishnan et al. [103] | 381 patients undergoing TAVR | Sarcopenia of the psoas muscles was associated with an increased risk of mortality in univariate (HR 2.3, P = 0.01) and multivariate (HR 2.5, P = 0.01) analysis. | Yes |
2020, Tzeng et al. [104] | 182 patients undergoing TAV | Patients with prolonged LOS had a higher STS score (P < 0.001) and significantly lower PMD (P < 0.001) than those with LOS ≤ 14 days. Neither PMA (P = 0.123) nor PMI (P = 0.271) was associated with prolonged LOS. | Yes |
2020, Michel et al. [47] | 1731 patients undergoing TAVR | Unadjusted survival by PMA quartile was significantly different in men (log rank P = 0.041) but not women (P = 0.099). In Bonferroni-adjusted multivariate analysis, PMA quartiles were not significantly associated with mortality. | No |
2020, Koizia et al. [105] | 60 patients undergoing TAVR | Psoas-lumbar vertebral index was 0.61, with a lower value in the frail group. There was no statistically significant correlation between the psoas measures, mean Reported Edmonton Frail Scale (REFS) score and mortality. A statistically significant relationshi p between the psoas size and REFS score (P = 0.043) was found. | Yes |
2020, Waduud et al. [45] | 420 patients undergoing TAVR | Standardised measurements of the PMA were not associated with either postintervention morbidity or mortality. There were no significant correlations between TPMA and any of the traditional frailty tools. | No |
2021, Uchida et al. [106] | 71 patients undergoing TAVR | PMI was calculated as volume/height2 (cm3 m–2). According to the gender-specific median value of PMIs, the enrolled patients were divided into two groups. The proportion of MACE-free survival was significantly lower in the low PMI group (log-rank P = 0.033), mainly due to the difference of hospital readmission for congestive heart failure. On multivariate Cox proportional hazard analysis, PMI remained an independent negative predictor of MACE (HR 0.95, P = 0.002). | Yes |
2021, Furzan et al. [107] | 245 patients undergoing TAVR. | Patients with PI (PMA/BSA) < 4 cm2 m–2 and psoas muscle density PD < 25 Hounsfield units (HU) were less likely to survive and to be discharged home compared with patients with PI ≥ 4 cm2 m–2 or PD > 25 HU. After repeating the propensity score matching with PI as a covariable, PD remained associated with mortality (90 days: OR 4.59; P < 0.001, 1 year: OR 6.14; P = 0.01, 3 years: OR 4.55; P = 0.03). | Yes |
2021, Walpot et al. [30] | 94 patients undergoing TAVR | PMD (mean CT attenuation coefficient of the psoas muscle (Psoas mean HU), low-density muscle (LDM) (0–29 HU) and high-density muscle (HDM) (30–100 HU)) was measured. Compared with patients with the lowest LDM (%), patients in the third and second tertiles had an increased hazard ratio for mortality (HR 2.871 and HR 5.044, respectively) in a multivariable model with EuroSCORE II, Barthel frailty index and LDM (%) (P = 0.231, 0.097 and 0.019, respectively). HDM/LDM and Psoas mean HU (as continuous variable) were also independent predictors of all-cause mortality (P = 0.019, P = 0.013, respectively). | Yes |
B. Post-CABG or implantation of an LVAD assist device. | |||
2016, Herbertson et al. [48] | 333 patients who underwent implantation of an LVAD. | – The primary outcome of inpatient death or prolonged length of stay occurred in 81% and 60% of patients with CT measured PMA sarcopenia and non-sarcopenia, respectively (P = 0.043). – A trend toward prolonged length of stay in sarcopenic patients but no difference in overall mortality was observed. | Yes |
2016, Zuckerman et al. [52] | 82 patients undergoing cardiosurgical procedures | Postoperative LOS was correlated with PMA (R = –0.47, P = 0.004). After adjustment for the predicted risk of prolonged length of stay (LOS), age, sex, and body surface area, PMA remained significantly associated with LOS (β = –2.359). | Yes |
2018, Hawkins et al. [53] | 240 patients after aortic valve replacement | – Patients were stratified by sarcopenia status, defined as PMI < 25th sex-specific percentile. – Patients with sarcopenia had higher 1-year mortality (31.9% versus 16.9% P = 0.03). PMI significantly predicted risk-adjusted 1-year mortality (OR 0.84, P = 0.02) and long-term mortality (HR 0.92, P = 0.04), as well as risk-adjusted major morbidity. | Yes |
2019, Okamura et al. [54] | 428 patients undergoing heart valve surgery | Multivariable analysis: PMA, as a marker of sarcopenia, was an independent predictor for decreased survival (HR 2.22; 95% CI: 1.26-3.92; P = .006). | Yes |
2019, Lopez et al. [49] | 160 patients with heart failure | Sarcopenia, defined as low skeletal muscle mass measurement, had 4.5 times the risk of all-cause mortality at 1 year compared to the rest of the cohort (P = 0.0016) after adjusting for significant covariates. | Yes |
2019, Yamashita et al. [55] | 664 patients undergoing cardiovascular surgery | Psoas muscle attenuation (MA) was measured as metrics of sarcopenia (low MA defined as below median). On multivariate Cox regression analysis, sarcopenic obesity was associated with increased risk of mortality after adjusting for EuroSCORE (HR 3.04). | Yes |
2019, Kurumisawa et al. [56] | 138 Haemodialysis-dependent patients who underwent cardiac surgery | According to the PMI, the study population was divided into the sarcopenia group and the non-sarcopenia group. Overall, in-hospital mortality did not differ between the sarcopenia and non-sarcopenia groups. Sarcopenic patients had significantly decreased long-term survival. Multivariate analysis revealed that low PMI was an independent predictor of long-term mortality (HR 1.92; P < 0.01). | Yes |
2020, Yamashita et al. [108] | 1068 patients with CVD undergoing surgery | Reduced psoas muscle density (PMD) was associated with postoperative mortality and added information prognostic for mortality to the existing prognostic model in CVD patients. | Yes |
2020, Okamura et al. [109] | 304 patients undergoing elective isolated off-pump CABG | Sarcopenia was defined as the lowest sex-specific quartile of the PMI (PMA normalised for height). Multivariable analysis showed that preoperative sarcopenia was an independent predictor of late mortality (HR 4.25; P < 0.001). Preoperative sarcopenia was not associated with major adverse cardiac and cerebrovascular events during follow-up. | Yes |
2020, Taniguchi et al. [57] | 56 patients undergoing aortic valve replacement (AVR) | A smaller indexed erector spinae muscle (ESM) cross-sectional area (per 1 cm m–2, OR: 0.71; P < 0.01) was identified as an independent predictor for prolonged hospitalization. Indexed PMA was not statistically significant. | Yes |
2020, Kiriya et al. [58] | 412 patients undergoing cardiac surgery | A multivariable analysis showed that low TPI and high intra-muscular adipose tissue content (IMAC) significantly predicted more major complications than other combinations (OR 2.375; P = 0.036, OR 3.973; P = 0.001). | Yes |
2020, Matsumura et al. [110] | 210 consecutive patients aged ≥ 60 years with acute heart failure | HFpEF patients with reduced PMI had significantly higher cardiac death rates than those with preserved PMI (38% vs. 16%, log-rank test P = 0.006). HFrEF patients had comparable cardiac death rates regardless of their PMI group (log-rank test P = 0.24). The multivariate Cox model revealed that patients with reduced PMI had a 2.3-fold higher risk of cardiac death compared with patients with preserved PMI (P = 0.01). | Yes |
Matsumoto, et al. [51] | 132 consecutive patients with non-ST-segment–elevation myocardial infarction | The multivariate Cox proportional hazards model revealed that reduced PMI was a statistically significant predictor of cardiovascular events (HR 3.30; 95% CI: 1.70–6.40; P < 0.001). | Yes |
2021, Funamizu et al. [50] | 392 patients after acute decompensated heart failure (ADHF) | Multiple linear regression analysis revealed that female sex, BMI, and E/e’, but not LVEF, were independently associated with PMI (cm2 m–2). Kaplan-Meier analysis showed low PMI was associated with higher rate of composite endpoint of all-cause death and ADHF re-hospitalization (P = 0.033). Cox proportional hazard model analysis identified that low PMI, but not BMI, was an independent predictor of the composite endpoint (HR 1.52, P = 0.024). | Yes |
2021, Yamashita et al. [59] | 853 patients who underwent cardiovascular surgery | Low skeletal muscled density (SMD) based on transverse abdominal CT images was defined as a mean Hounsfield unit of the psoas muscle < 45. Low SMDs combined with each definition of muscle dysfunction had the highest risk of all-cause death (HR: lowest 3.666 to highest 6.002), and patients with neither low SMDs nor muscle dysfunction had the lowest risk of all-cause and cardiovascular-related events. | Yes |
1. Assessment of PMA in patients undergoing TAVR. According to the ESC guidelines, TAVR is an appropriate treatment for severe symptomatic aortic stenosis in older patients (≥ 75 years), or in those who are high risk or unsuitable for surgery [43]. Frailty assessment is mandatory for candidates for a TAVR procedure. A study demonstrated that low PMI was associated with all-cause and cardiac mortality in females. The 1-year adjusted cardiac mortality HRs in females in the high-PMI and mid-PMI range were 0.40 and 0.14, respectively [31]. Similar effects were observed for 2-year and 30-day cardiac and all-cause mortality. When PMI was added to surgical risk scores, the predictive value for 1-year mortality was improved in females [31].
Another study, including 1076 patients undergoing TAVR, demonstrated that PMI at level L4 (HR 0.083, P < 0.001) was independently associated with mortality during the follow-up. Adding PMI to the STS score resulted in a net reclassification improvement for cumulative mortality [44].
A study including 94 patients undergoing TAVR with a median follow-up of 5 years showed that LDM (%), defined as the psoas muscle fraction with muscle attenuation ranging from 0 to 29 HU, was an independent predictor of all-cause mortality after TAVR in a multivariable model with EuroSCORE II and the Barthel frailty score. Compared to patients in the first LDM (%) tertile, patients in the third and second tertile had a hazard ratio (HR) of 2.871 and 5.044, respectively [30].
Of the 17 studies searching for a correlation between outcomes after TAVR and PMI (or PMD), only three studies failed to demonstrate a significant association [45–47].
2. Assessment of PMA in other cardiac conditions. Herbertson et al. observed that in patients undergoing left ventricular assist device (LVAD) implantation, the composite primary outcome of prolonged stay and inpatient death occurred in 81% and 60% of patients with PMI measured sarcopenia and non-sarcopenia, respectively (P = 0.043). A trend toward a prolonged stay was observed in sarcopenic patients, but no difference in overall mortality was seen [48]. Another study, including 160 patients with heart failure, showed that sarcopenia, defined as low SMI, was associated with a 4.5-fold risk of all-cause mortality at 1 year compared to the rest of the cohort (P = 0.0016) [49]. Funamizu et al. found that low PMI was a predictor of adverse outcomes (mortality and rehospitalisation rate) in patients after the first episode of acute heart failure (HR 1.52, P = 0.024) [50].
In patients suffering from a non-ST elevation myocardial infarction, a reduced PMI was a statistically significant predictor of cardiovascular events (HR 3.30; P < 0.001) [51].
Several studies have found associations between PMI and outcome after CABG or valve surgery, with low PMI predicting unfavourable outcomes [52–59].
B. PMA assessment in patients with peripheral vascular disease
A study including 135 patients undergoing endovascular aortic replacement (EVAR) demonstrated that the 5-year mortality was highest in patients with the lowest PMA tertile (odds ratio [OR]: 3.9) [60]. Another study, including 407 patients undergoing EVAR, obtaining similar results, revealing that lower PMA was associated with decreased survival (adjusted HR = 1.68; P = 0.006) [61].
Pizzimenti et al. demonstrated that sarcopenia, defined as PMI < 5.5 cm2 m–2 in men and < 4.0 cm2 m–2 in women, was associated with significantly increased 30-day mortality and 30-day morbidity compared to the non-sarcopenic group (P = 0.015 and P < 0.001, respectively) in patients with critical limb-threatening ischaemia [62].
Gomibuchi et al. conducted a study that included 324 patients with acute type A aortic dissection undergoing aortic replacement. The 5-year survival was significantly worse for the group of patients with intramuscular fat (IMF) deposition (IMF 73.8% vs. non-IMF 88.2%; P = 0.010). The multivariable Cox analysis showed that IMF deposition was a statistically significant predictor of poor survival (HR = 3.26; P = 0.004), unlike PMI [63].
Supplemental Table 1 lists the studies that searched for associations between PMI and outcome in patients with peripheral vascular disease.
C. PMA assessment in patients with trauma
Several studies have demonstrated an association between CT-measured PMI and outcome after trauma. Touban et al. found in a series of 558 elderly patients that small PMA was associated with increased 1-year mortality (HR = 0.93, P < 0.0001). Sex stratification revealed a statistically significant mortality HR of 0.89 (P = 0.002) in male but not in female patients (P = 0.103) [64].
A study including 405 trauma patients aged ≥ 65 years old and with Injury Severity Scores above 15 showed that sarcopenia, determined by measuring PMI, was significantly associated with higher mortality (OR = 2.20; P = 0.027) [65].
Cho et al. performed a study that included 179 patients with brain injury. It was found that PMI was statistically significant as a risk factor for mortality (P = 0.023; OR = 0.715). A statistically significant correlation was found between PMI and Glasgow Outcome Scale (GOS) score (correlation coefficient, 0.168; P = 0.003) [66].
Supplemental Table 2 lists the studies searching for associations between PMI and other CT-measured anthropomorphic variables and outcomes in trauma patients.
D. PMA assessment in patients in the ICU/Emergency Department
This section will discuss studies that searched for CT-acquired sarcopenia variables and the outcome of patients in the Emergency Department and ICU. The selection of the studies in this section required that authors explicitly focus on staying in the Emergency Department or the ICU. Fifteen studies were found, and their main results are listed in Table 6.
TABLE 6
Psoas muscle area and/or attenuation variables and outcome after Emergency Department/ICU
Year & Reference | Number of patients | Study results | Association (Y/N) |
---|---|---|---|
2017, Rangel et al. [111] | 297 patients with emergency abdominal surgery patients and available preoperative imaging and height | CT measured sarcopenia was independently associated with increased in-hospital mortality (risk ratio: 2.6; 95% CI: 1.6–3.7) and mortality at 30 days (HR 3.7; 95% CI: 1.9–7.4), 90 days (HR ± 3.3; 95% CI: 1.8–6.0), 180 days (HR 2.5; 95% CI: 1.4–4.4), and 1 year (HR 2.4; 95% CI: 1.4–3.9). | Yes |
2018, Trotter et al. [112] | 259 patients after emergency laparotomy | – Sarcopenia was assessed by PMA and psoas density on preoperative CT. – Sarcopenia measured by psoas density was associated with increased mortality compared with patients who did not develop sarcopenia at 30 days (P < 0.001; OR 4.42) and at 1 year (P < 0.001; OR 6.05). An increase in mortality was seen in patients with sarcopenia measured by PMA at 30 days (OR 2.71; P = 0.013) and at 1 year (OR 2.82, P < 0.001). | Yes |
2018, Lee et al. [69] | 274 patients with sepsis | Muscle mass depletion measured by PMA was independently associated with 28-day mortality on multivariate logistic analysis (OR 2.79; P = 0.01). | Yes |
2018, Francomacaro et al. [113] | 967 patients with urgent exploratory laparotomy | Multivariate analysis found that patients in the lowest quartile of PMA had higher mortality and complication rate, were less likely to be discharged home, were more likely to undergo unplanned re-operation, and had a longer length of stay. | Yes |
2019, Brandt et al. [114] | 150 elderly patients undergoing emergency abdominal surgery | Sarcopenic patients had a higher 90-day mortality (60.5%) than non-sarcopenic patients (36.6%) (OR 2.66, P = 0.01). Multivariate logistic regression: sarcopenia was independently associated with 90-day mortality. | Yes |
2019, Hamidi et al. [115] | 152 patients undergoing emergency general surgery | Sarcopenia was defined as the lowest sex-specific quartile of PMI. On regression analysis, sarcopenia was an independent predictor of minor complications (OR 1.8) and discharge to rehab (OR 1.9) No association with major complications, mortality, 30-day complications, readmissions, and mortality was found. | Yes |
2019, Kou et al. [116] | 96 patients undergoing mechanical ventilation in the surgical intensive care unit (ICU) | Multivariate logistic regression: sarcopenia was an independent risk factor for difficult-to-wean (DtW) and ICU mortality. The AUC of ROC curve of PMA for predicting successful weaning was 0.727 and 0.720 in female and male patients, respectively. After combining MPA and conventional weaning parameters, the AUC for DtW increased from 0.836 to 0.911 and from 0.835 to 0.922 in female and male patients, respectively. | Yes |
2020, Zhang et al. [117] | 96 critically ill surgical patients | PMA is a protective factor for ICU mortality (OR 0.99, P = 0.0269). Sarcopenia, based on PMA assessment, increased the risk of ICU mortality (OR 3.73, P = 0.0167). Discrimination of ICU mortality was significantly higher using SOFA (AUROC, 0.7810) than either PMA (AUROC, 0.7023) or APACHE II score (AUROC, 0.7447). The combined PMA with SOFA ROC (AUROC, 0.8647) was the highest when compared to the models. | Yes |
2020, Simpson et al. [68] | 264 older adults underwent emergency laparotomy | Psoas major was measured at the L3 level and normalised to height (psoas muscle index, PMI), L3 vertebral body (psoas muscle: L3 ratio, PML3) or body surface area (psoas: body surface area, PBSA). A multivariate analysis confirmed each method of psoas major calculation (P < 0.0001) to be associated with mortality. PML3 is superior to PMI or PBSA. | Yes |
2020, Akkoc et al. [118] | 89 patients with pulmonary embolism requiring ICU stay | The increase in the value of psoas muscle area is associated with a decrease in the rate of in-hospital mortality. In patients with in-hospital mortality related to pulmonary embolism, the higher PESI and the lower value of psoas muscle area were considered in accordance with the outcome of patients. | Yes |
2021, Hung et al. [70] | 99 adult patients, undergoing an abdominal contrast CT scan in the ED with the final diagnosis of splenic abscess | PMA normalised for height was used to determine sarcopenia. Splenic abscess patients with sarcopenia were 7.56 times more at risk of in-hospital mortality than those without sarcopenia (multivariate-adjusted HR 7.56). Presence of sarcopenia was found to have 84.62% sensitivity and 96.49% negative predictive value in predicting mortality. | Yes |
2021, Malle et al. [119] | 37 critically ill patients from the VITdAL-ICU trial with available abdominal CT scans | No significant influence on hospital length of stay or on 6-month mortality was found, irrespective of the morphometric parameter used (PMA, PMD, skeletal muscle index [SMI], bone mineral density [BMD]) (P > 0.05). | No |
2020, Kobori et al. [67] | 39 ICU patients | PMA and PMI were greater in the extubation success group than in the extubation failure group (P = 0.022 and P = 0.032, respectively). | Yes |
2021, Tee et al. [120] | 1192 elderly patients (aged ≥ 65 years) undergoing abdominal surgery | CT-measured muscle index (MI) and muscle gauge (MG) values were used. A low L4 psoas MG (PMG) value was an independent risk factor for overall complications and mortality in elderly patients with abdominal emergencies. | Yes |
2021, Dreydemy et al. [121] | 162 critically ill trauma patients without chronic renal dysfunction | Both ΔPMA and %ΔPMA were higher in augmented renal clearance (ARC) patients (P = 0.010 and P = 0.008, respectively). Critically ill trauma patients with ARC are characterised by a lower nitrogen balance and increased muscle loss over the 10 first days after ICU admission. | Yes |
Kobori et al. reported that PMA and PMI were larger in the extubation success group than in the extubation failure group (P = 0.022 and P = 0.032, respectively) [67].
A study including 264 older adults who underwent emergency laparotomy showed that PMI, PML3 (vertebral body : psoas muscle ratio at level L3), and PBSA (PMA indexed to body surface area) were all associated with mortality (P < 0.001), but PML3 was superior to PMI or PBSA [68].
Lee et al. included 274 patients with sepsis and found that muscle mass depletion, determined by PMA measurements, was independently associated with 28-day mortality (OR = 2.79; P = 0.01) [69].
Hung et al. analysed 99 adult patients with a documented splenic abscess on abdominal contrast CT scan. PMI was measured to determine sarcopenia. Splenic abscess patients with sarcopenia were 7.56 times more at risk of in-hospital mortality than those without sarcopenia (adjusted HR = 7.56). Sarcopenia has a sensitivity of 84.62% and a negative predictive value for mortality of 96.5% [70].
E. PMA assessment in patients undergoing elective orthopaedic surgery
Studies have demonstrated that CT-acquired skeletal muscle anthropomorphic measurements predict the outcome after orthopaedic surgery.
A study including 395 patients undergoing lumbar surgery showed that female and male patients with the lowest PMA tertile had an OR of 1.70 (95% CI: 1.04–2.79, P = 0.035) and 2.42 (95% CI: 1.17–5.01, P = 0.016) for postoperative complications and mortality, respectively [71].
Another study, examining 123 patients who had undergone single-level lateral lumbar interbody fusion, revealed that PMI was a predictor for achieving a substantial clinical benefit (SCB) in males and females (OR = 1.251; P = 0.038 and OR = 1.795; P = 0.023, respectively). The optimal thresholds of PMI for an SCB were 8.18 cm2 m–2 for males and 4.43 cm2 m–2 for females [72].
Supplemental Table 3 lists the studies involving patients undergoing elective orthopaedic surgery and summarises the primary outcome results regarding the value of PMI and other CT-acquired musculoskeletal variables to predict postoperative adverse events.
F. PMA in the assessment of patients undergoing transplantation
Supplemental Table 4 lists the 33 available studies examining the potential role of PMI/SMI in the risk grading of transplant candidates. Especially in liver transplantation (LT), many studies have sought correlations between PMI/SMI and outcomes after LT.
Hammand et al. performed a study including 200 patients after adult-to-adult living donor liver transplantation and found that low PMI was associated with significant postoperative complications, postoperative bacteraemia, and poorer overall survival (OS) (P < 0.001) [73].
Another study, examining 115 patients after living donor liver transplantation due to cirrhosis, revealed that SMI was a predictor of 90-day mortality (OR = 0.83; P = 0.016) [74].
The study of Wu et al., including 271 living-donor liver transplant (LDLT) recipients, used a PMI cut-off value of 2.63 cm2 m–2 as the threshold for sarcopenia. The female cohort demonstrated that the 1-, 3-, 5- and 10-year overall survival rates were significantly better in the non-sarcopenia group than in the sarcopenia group (P = 0.041) [75].
In a consensus document, the Japan Society of Hepatology proposed a definition of sarcopenia by recommending a threshold for PMI of 6.36 cm2 m–2 in males and 3.92 cm2 m–2 in females [76].
In a study including 95 patients with primary lung transplantation, the adjusted Cox analysis found an increased hazard for all-cause mortality (HR = 5.8, P < 0.01) and graft failure (HR = 14.7, P < 0.01) in sarcopenic patients [77].
G. PMA in assessing patients undergoing major non-cardiovascular and non-oncological surgery
A study including 152 consecutive patients who underwent open pancreatoduodenectomy showed that the rate of significant complications was associated with PMI (OR = 0.605, P = 0.009), PMI-defined sarcopenia (OR = 8.256, P = 0.000), and sarcopenic overweight/obesity (OR = 7.462, P = 0.002) [78].
Lo et al. performed a study including 100 patients after enterocutaneous fistula repair. They defined the lowest interquartile HU attenuation coefficient (HUAC) as the threshold for low muscle quality (LMQ). LMQ was associated with 1-year leak (P < 0.005), 1-year mortality (RR = 2.22, P < 0.04) and 3-year mortality (RR = 2.13, P < 0.007), complication risk (P < 0.001), and dependent discharge (P < 0.004) compared to patients without LMQ [79].
Another study demonstrated that PMI-measured sarcopenia is an independent predictor of a gastric leak [80]. Supplemental Table 5 summarises all studies.
H. PMA in the assessment of patients undergoing oncologic surgery
Supplemental Table 6 lists 72 studies examining the impact of skeletal muscle volume on outcomes in patients undergoing oncological surgery and reports the main results of these studies. Given the preoperative tumour staging process, abdominal CT axial slices, allowing PMI and SMI measurements, are available in these patients.
A meta-analysis including 53 studies (14 295 patients) of patients undergoing oncological surgery analysed the impact of the skeletal muscle area (SMA) on the clinical outcome. Compared to measuring the total SMA, PMA measurements reported a lower prevalence of sarcopenia, 43% versus 33%, respectively [81]. Preoperative sarcopenia was associated with an increased risk of severe postoperative complications (OR = 1.44, P < 0.001) and 30-day mortality (OR = 2.15, P < 0.001). A low PMA was a stronger predictor for severe postoperative complications than a low SMA (OR = 2.06 and OR = 1.32, respectively) and 30-day mortality (OR = 6.17 and OR = 1.80, respectively). This effect was independent of tumour location [81].
Deng et al. reviewed the medical literature on patients surgically treated for non-small cell lung (NSCLC) cancer and concluded that sarcopenia could be used as a predictor of poor prognosis in surgically treated NSCLC patients [82].
I. PMA in the assessment of patients with internal diseases
Supplemental Table 7 lists the studies examining the impact of skeletal muscle volume on outcomes in patients with internal diseases and reports the main results of these studies. Most studies were performed on patients with malignancies or end-stage liver disease (ESLD).
A systematic meta-analysis, including 20 eligible studies, examined the impact of sarcopenia on outcome in liver cirrhosis. 13 studies enrolled Western participants, and the remaining seven included Asian participants. This study reported a prevalence rate of sarcopenia of 48.1%. Sarcopenia was more prevalent in males (61.6%) than in females (36%). Poorer survival rates and an increased risk of complications, such as infection, were observed in sarcopenic patients compared to non-sarcopenic patients. Furthermore, Asians had higher mortality related to sarcopenia compared to Western participants [83].
A meta-analysis of 19 studies (1763 patients with cancer treated with immune checkpoint inhibitors) demonstrated that patients with sarcopenia at pre-immunotherapy had poorer overall survival (OS) and progression-free survival (PFS) than those without sarcopenia. Furthermore, sarcopenic patients had worse objective response rates (ORR) (P = 0.001) and disease control rates (DCR) [84]. Sarcopenia, determined by measuring SMI, was associated with worse ORR, DCR, PFS, and OS than patients without sarcopenia. However, patients with sarcopenia, determined by measuring PMI, had poor ORR and DCR. However, PMI measured sarcopenia was no prognostic factor for PFS and OS [84].
J. PMA assessment in the paediatric population
Supplemental Table 8 lists the studies searching for an association between CT-measured anthropomorphic variables and outcomes in severely ill children. The global trend, seen in adults, seems to be confirmed in the paediatric populations, with most studies indicating that low PMI and SMI are associated with unfavourable outcomes.
A study included 101 children in the workup for neuroblastoma and demonstrated that preoperative sarcopenia was associated with reduced 5-year survival after surgery, with an HR of 4.18 [85].
CONCLUSIONS
Sarcopenia has been associated with poor survival, prolonged hospital stays, and higher complication rates in patients with severe medical conditions.
Anthropomorphic assessment can be performed by measuring the PMI at the third lumbar vertebra level. Many frail patients admitted to the Emergency Department or hospitalised in the ICU need to undergo abdominal CT studies for various medical reasons. PMA and SMA can be quantified in these patients by postprocessing the acquired images on a dedicated workstation without extra radiation exposure or dye administration.
The psoas muscle quality can be assessed by measuring the psoas muscle CT attenuation coefficient.
Extensive research has proven the utility of CT-measured PMI and PMD to predict survival, complications, and length of stay in the ICU in critically ill patients with various underlying medical conditions, such as severe chronic diseases, cancer, liver cirrhosis, traumatic injury, and cardiovascular diseases.
Further standardization of the CT measured methods to quantify PMI, SMI, and PMD is needed. Notwithstanding these limitations, PMI and PMD seem promising new biomarkers to obtain additional prognostic information in patients undergoing abdominal CT for various medical reasons. Reliable semi-automated and automated software quantifying CT-acquired PMA and PMD has been developed, enabling the use of these new biomarkers in the clinical setting.