Introduction
Heart failure (HF) is a clinical syndrome induced by structural or functional cardiac abnormalities [1]. HF symptoms include dyspnoea and fatigue, often accompanied by typical physical signs, such as pulmonary rales, distended jugular veins, peripheral oedema, or more recently, B-lines on lung ultrasound [2, 3]. Coronary artery disease and hypertension are the most common causes of HF in developed countries [4]. Other causes include valvular diseases, arrhythmias, congenital heart diseases, cardiomyopathies, infections (e.g. myocarditis, endocarditis), metabolic diseases, drug-induced HF, etc. [1]. In developed countries, HF has become an essential public health problem affecting 2% of adults. The number of hospital admissions attributed to HF has tripled since the 1990s, amounting to 1.25 million in 2018 in the USA [5, 6]. In Poland, there are up to 750,000 people living with HF, and 194,000 hospitalizations in 2017 were due to HF, according to the National Health Fund [7]. It is predicted that these numbers will increase [6].
Acute HF (AHF) is a sudden, life-threatening condition, defined as a gradual or rapid onset of symptoms and/or signs of HF. Patients with AHF require urgent medical attention [1]. From a clinical perspective, AHF is divided into de novo HF, where symptoms and signs occur in patients with no previous history of HF, and acutely decompensated HF (ADHF), where symptoms and signs exacerbate in patients with previously diagnosed chronic HF [2]. AHF is the most frequent cause of unplanned hospital admission in patients above 65 years of age, associated with 4% to 12% in-hospital mortality [5, 6] and 21% to 35% 1-year mortality post discharge [8].
Considering the serious prognosis in AHF patients, it is very important to understand the mechanisms and haemodynamic status in an individual AHF patient, thus preventing end-organ failure and death. Haemodynamic monitoring is a serial assessment of cardiovascular function, intended to detect physiological abnormalities at the earliest stages, determine which interventions could be most effective, and provide the basis for initiating the most appropriate therapy and evaluate its effects. In this article, we aimed to provide the pathophysiological explanation of the physical terms and parameters used for haemodynamic monitoring in AHF, and to summarize the working principles, advantages, and disadvantages of the currently used methods of haemodynamic monitoring.
Clinical presentations of acute heart failure
To understand the meaning of various parameters used for haemodynamic monitoring in AHF, it is crucial to describe the clinical presentations of AHF. There are 4 major clinical presentations of AHF, mainly based on the presence of signs of peripheral hypoperfusion (decreased delivery of oxygen to tissues) and/or congestion (excess fluid in an intravascular compartment in the lungs): (i) acute decompensated heart failure, (ii) acute pulmonary oedema, (iii) isolated right ventricular failure, and (iv) cardiogenic shock [1]. Patients may be also described as ‘wet’ vs. ‘dry’ (if congestion is present vs. absent) and as ‘cold’ vs. ‘warm’ (if hypoperfusion is present vs. absent), according to the Forrester classification established in the 1970s [9].
Acute decompensated heart failure (ADHF) is the most common AHF presentation [8]. Most patients admitted to the hospital with ADHF (80–85%) experience an exacerbation of chronic HF, whereas only 15–20% are diagnosed with HF de novo [10]. Left ventricle (LV) dysfunction, as well as sodium and water renal retention, compose the main mechanism of ADHF. They lead to accumulation of fluid in pulmonary and systemic circulation, and an increase of left- and right-heart filling pressure. Consequently, the patient usually presents with weight gain, exertional dyspnoea or orthopnoea, and pulmonary and/or systemic congestion.
Acute pulmonary oedema is usually induced by decompensated valvular heart disease, increased afterload, or diastolic LV dysfunction (in heart failure with preserved ejection fraction, HFpEF), which induces fluid redistribution to the lungs and acute respiratory failure. Patients present with acute dyspnoea, orthopnoea, tachypnoea (respiratory rate > 25 breaths/min), and increased work of breathing [11]. The course of acute pulmonary oedema is much more rapid than ADHF [12]. Pulmonary oedema can be quickly and noninvasively confirmed with the use of lung ultrasound (LUS), being visible as multiple B-lines [3].
Isolated right ventricular failure occurs due to right ventricular (RV) dysfunction or pulmonary hypertension. Increased central venous pressure (CVP) and systemic congestion along with LV filling impairment lead to reduced systemic cardiac output (CO) and therefore systemic hypoperfusion [13].
Cardiogenic shock is a consequence of severe cardiac dysfunction, including inadequate CO, life-threatening hypoperfusion, and hypotension. This can result in multiorgan failure and death [14]. Acute myocardial infarction accounts for 81% of cases of cardiogenic shock [15].
Haemodynamic monitoring
The purpose of properly functioning circulation is to supply tissues/organs with oxygen and nutrients. If the heart pump function is suddenly impaired, it is crucial to quickly identify the problem and provide an appropriate therapy to improve organ perfusion. Haemodynamic monitoring is a serial assessment of cardiovascular function, intended to detect physiological abnormalities at the earliest stages, when interventions could be most effective, and to provide the basis for starting a correct therapy and evaluating its effects [16]. It may be useful in differentiating the type of shock, which is essential to initiate an appropriate therapy [17]. The physical examination and the use of standard haemodynamic variables, such as heart rate, blood pressure (BP), and urine output, are essential components of the clinical assessment. However, objective measures of haemodynamics are crucial for making a prompt and accurate evaluation [18]. Over the past decades, haemodynamic monitoring techniques have greatly evolved. Currently, they range from very invasive to non-invasive, from intermittent to continuous, and in terms of the provided haemodynamic parameters.
Invasive techniques include pulmonary artery catheterization and transpulmonary thermodilution. Minimally invasive techniques include oesophageal Doppler and noncalibrated pulse wave analysis. Non-invasive techniques contain echocardiography, bioimpedance, and bioreactance techniques as well as non-invasive pulse contour methods [17]. Each of these techniques has specific indications and limitations (Table I) [19–47]. Invasive methods are considered more accurate, providing results that are more reproducible and closer to the actual condition. However, they are associated with an increased rate of complications (5–10% in PAC and ca. 7% in thermodilution) [19, 20]. Non-invasive methods usually allow less accurate measurements but are generally easier to apply. Thus, the choice of the haemodynamic monitoring method depends on the clinical situation of the patient and the experience of the medical staff. Below, we provide information on the most common methods that may support the selection of the appropriate method in clinical practice. The terms and definitions used in hemodynamic monitoring are summarized in Table II, and a summary of the methods of haemodynamic monitoring is listed in Table I [21–39].
Table I
Summary of the key information on the methods of haemodynamic monitoring
Method | Invasiveness | Working principle | Indication | Advantages | Disadvantages |
---|---|---|---|---|---|
Echocardiography Jozwiak et al. 2018 [40] De Backer et al., 2015 [17] Lancellotti et al., 2015 [41] Flower et al., 2021 [42] | – | Imaging of structure and functioning of the heart with the use of an ultrasound probe applied to the chest wall | First-line point-of-care imaging in shock suspicion, evaluation of cardiac contractility, confirmation of diagnoses made during other tests | Non-invasive, easily accessible wide range of provided parameters | Required experience and training, operator-dependant, challenging cardiac views (subcostal window) |
Bioimpedance and bioreactance analysis Jakovljevic et al., 2014 [43] | – | This method measures a different response of tissues to an electric current flow, which provides real-time flow information | Guiding fluid therapy in hemodynamically unstable patients Quick evaluation of response to fluid therapy in the passive leg raise test | Fully non-invasive method Provides continuous measurement of CO | Low accuracy, especially for bioimpedance |
Pulse contour analysis Saugel et al., 2021 [44] | ± | Evaluation of CO based on continuous analysis of the arterial pressure waveform | Management of fluid therapy and drug administration during hemodynamic instability | Provides continuous measurement of CO. Can be fully non-invasive or minimally invasive | Low accuracy if not calibrated by other methods. Requires blood flow with a relevant pulse wave |
Oesophageal Doppler Mateu Campos et al., 2012 [45] Hamzaoui et al., 2015 [46] | + | Estimation of aortic blood flow with the use of Doppler probe emitting ultrasound waves, placed in the oesophagus | Prediction of fluid responsiveness. Provides information on cardiovascular state of the patient | Minimally invasive. Alternative to thermodilution in measuring of CO | Risk of malposition. Estimated parameters may be incorrect when MAP changes rapidly |
Pulmonary artery catheterization De Backer et al., 2015 [17] Cecconi et al., 2014 [47] | ++ | Assessment of “right heart’s” parameters with the use of a catheter inserted through the central access | Refractory shock with RV dysfunction gold standard in CO measuring evaluation and diagnosis of PAH differentiating cardiogenic shock from non-cardiogenic shock | Wide range of provided parameters, impact on management and clinical outcome | Invasive. Unable to constantly track CO |
Transpulmonary thermodilution Monnet et al., 2017 [20] | ++ | Assessment of CO based on the time it takes to detect a change in arterial blood temperature after injection of cold fluid into the central vein | Acute circulatory failure with no response to the initial therapy | Reference method for evaluating CO. Can be used to calibrate continuous non-invasive methods. Ability to measure other parameters than CO (GEDVI, EVLWI, PVPI) | Invasive method. Many factors can interfere with the measurement |
Table II
Terms and definitions used in haemodynamic monitoring
Term | Definition |
---|---|
Cardiac output (CO) | The amount of blood pumped every minute by the heart (CO = HR × SV). CO in adults is generally 5–6 l/min at rest, to more than 35 l/min in elite athletes during exercise [21]. |
Stroke volume (SV) | Volume of blood pumped from the left ventricle during one cardiac cycle. Reference value is 70 ±14 ml/beat [22]. |
Afterload | Also called vascular resistance; the pressure that the heart must overcome to eject blood during contraction. It is proportional to the arterial pressure – as the arterial pressures increase, the afterload rises [23]. |
Preload | The stretch of cardiac muscle cells during the end of diastole (relaxation). Venous blood returning to the heart during diastole increases the volume of the ventricles. This causes the sarcomeres to stretch, thus increasing preload [24]. |
Hypotension | One definition is a SBP below 90 mm Hg and/or DBP below 60 mm Hg [11]. However, for the purpose of haemodynamic monitoring in the setting of AHF, the assessment of MAP is a more useful parameter. It correlates better with organ hypoperfusion. It can be calculated from the formula MAP = DBP + 1/3(SBP – DBP) or by using special appliances. Most commonly, it is necessary to maintain MAP above 60 mm Hg for normal perfusion [25]. |
Pulmonary artery catheter (PAC) | Also called a Swan-Ganz catheter; an intravenous catheter inserted through a central vein (e.g. femoral, jugular, axillary/subclavian) into the right side of the heart and to the pulmonary artery [26]. |
Cardiac index (CI) | A parameter that relates CO to the patient’s body surface area. Because CO varies with patient size, CI is an easier parameter to standardize than CO. The normal value for the cardiac index should be between 2.5 and 4.0 l/min/m2. A value under 2.0 should raise suspicion for cardiogenic shock [27]. |
Pulmonary capillary wedge pressure (PCWP), also called pulmonary artery occlusion pressure (PAOP) | A parameter used to assess LV filling and left atrial pressure. It is measured by inserting a Swan-Ganz catheter and advancing it into a branch of the pulmonary artery. The balloon is then inflated, which closes the branch of the pulmonary artery (and isolates the pressure from the one produced by RV) and then provides a pressure reading that corresponds with the pressure of the left atrium. The normal PCWP is between 4 and 12 mm Hg [28]. |
Left ventricular end diastolic pressure (LVEDP) | The pressure in the LV just before heart contraction. |
Right ventricular end diastolic pressure (RVEDP) | The pressure in the RV just before heart contraction. |
Central venous pressure (CVP) | The pressure obtained by the central venous catheter positioned in the SVC or IVC near the right atrium, or in the right atrium. CVP is often used as an assessment of haemodynamic status, particularly in the intensive care unit. The normal value of CVP for self-ventilating patients is 0–8 mm Hg [29, 30]. |
Mixed venous oxygen saturation (SvO2) | A measure of the oxygen content of the blood returning to the right side of the heart after perfusing the entire body. When the oxygen supply is insufficient to meet the metabolic demands of the tissues, an abnormal SvO2 reflects an inadequacy in the systemic oxygenation. Therefore, SvO2 is dependent on oxygen delivery and extraction. The most frequently used formula to calculate this parameter is SvO2 = (3 × SVC + IVC)/4. The normal value of SvO2 is 65–70% [31, 32]. |
Ejection fraction (EF)/left ventricular ejection fraction (LVEF) | A measurement (expressed in %) of how much blood in the LV was pumped out during one heart contraction. This is the ratio of SV to EDV, EF = SV/EDV × 100%. Normal EF may be between 50% and 70% [33]. |
Pulmonary artery pulsatility index (PAPi) | A measure of right heart function, derived from right atrial and pulmonary artery pulse pressures (formula: [systolic pulmonary artery (PA) pressure – diastolic PA pressure]/right atrial (RA) pressure). It reflects changes in any of the components of the right heart system [34]. It may also function as a predictor of adverse clinical events in patients with advanced HF [35]. |
Extravascular lung water (EVLW) | The amount of fluid accumulated in the lungs (in alveolar and interstitial spaces), which increases in conditions like lung oedema. It corresponds to the sum of interstitial, intracellular, alveolar, and lymphatic fluid, not including pleural effusions. It is suggested that normal values of EVLW should be < 10 ml/kg [36]. |
Pulmonary vascular permeability index (PVPI) | The ratio of EVLW to PBV, which shows the permeability of the barrier between alveoli and capillaries [37]. |
Global end-diastolic volume (GEDV) | The volumes of all 4 heart chambers at the end of diastole, just before heart contraction. A proposed reference value for the GEDV index is between 680 and 800 ml/m2 [38]. |
Cardiac function index (CFI) | An estimation of cardiac systolic function, defined as the ratio of CO/GEDV. The normal value of CFI is between 4.5 min–1 and 6.5 min–1 [39]. |
[i] AHF – acute heart failure, DBP – diastolic blood pressure, EDV – end-diastolic volume, HF – heart failure, HR – heart rate, IVC – inferior vena cava, LV – left ventricle, MAP – mean arterial pressure, PA – pulmonary artery, PBV – pulmonary blood volume, RA – right atrium, RV – right ventricle, SBP – systolic blood pressure, SVC – superior vena cava.
Echocardiography
Transthoracic echocardiography (TTE) is more of a haemodynamic evaluation technique rather than haemodynamic monitoring, because it cannot provide a continuous haemodynamic measurement. Nevertheless, it is recommended that TTE be performed in patients with suspected and/or symptomatic AHF [1]. It enables a non-invasive bedside assessment of cardiac structure and function (Figure 1 A) and detects the presence of congestion or a potential anatomical cause of AHF [41]. TTE assesses cardiac chamber size and its function, valvular structure and motion, the anatomy of the pericardial space, as well as an overall and regional systolic and diastolic function [48]. With the help of TTE, the type of shock can be quickly recognized [40].
Figure. 1
A – Diagram showing the principle of echocardiography. B – Schematic application of the bioimpedance and bioreactance analysis. Flowing blood and other body tissues cause different resistance to the flowing current. Analysis of changes in these values allows evaluation of CO. C – Diagram showing the principle of oesophageal Doppler effect
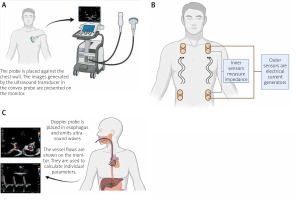
The 3 main TTE-based protocols in patients with AHF include the focused-assessed transthoracic echocardiography (FATE), focused assessment with sonography for trauma (FAST), and the rapid ultrasound for shock and hypotension (RUSH). FATE is a protocol that enables a focused and systematic approach in critically ill patients, aiming to find a potentially reversible cause of haemodynamic instability, such as pulmonary embolism, cardiac tamponade, systolic heart failure, aortic dissection, hypovolaemic shock, obstructive shock, or distributive shock [49, 50]. The FAST protocol is used to exclude tamponade in trauma patients when intra-abdominal or thoracic bleeding is suspected, whereas the RUSHed protocol is used to detect pathologies like tamponade, pneumo- and haemothorax, pulmonary embolism, aortic dissection, and cardiogenic shock that may be the cause of shock or hypotension [51]. TTE may be used to detect a possible cause of AHF and subsequently to initiate the appropriate treatment, and to optimize the previously initiated treatment.
TTE provides an estimation of stroke volume (SV) and hence CO [16]. Based on the CO, the amount of fluid or vasopressors administered intravenously can be adjusted. In conditions where CO is decreased (e.g. cardiogenic shock or LV systolic dysfunction), fluid resuscitation is required to increase CO and ensure oxygen delivery and organ perfusion. Enlarging the preload with intravenous fluids improves CO, but at some point the patient reaches a plateau and further administration of fluids results in clinical harm, such as tissue oedema, hypoxaemia, or even death. Thus, it is essential to perform regular TTE examinations to estimate whether fluid resuscitation is still needed [52].
Another important variable, obtained by TTE, is the left ventricular ejection fraction (LVEF). It depends on both LV contractility and afterload. However, it is not a precise marker of LV contractility; instead, it reflects the heart adaptation to the actual loading conditions. Therefore, when interpreting LVEF, systolic arterial BP must be taken into account. This is particularly important during shock, when LV afterload can markedly change over a short period [47]. Many authors also propose the use of TTE to improve the diagnosis of septic cardiomyopathy, e.g. by assessing ventricular contractility, dilations of ventricles, or filling pressures [53, 54].
TTE can be also used to diagnose pulmonary hypertension by measuring pulmonary artery pressure (PAP) with good accuracy [55]. These measurements are particularly indicated in cases of RV dysfunction. The gold standard for diagnosis is invasive right heart catheterization; this method will be described later [17].
TTE is also used to confirm diagnoses made while performing other tests [17, 40]. It is also speculated that the use of advanced TTE could improve diagnostic accuracy, reduce delays in treatment, shorten hospitalizations, and improve prognosis [56, 57]. Nevertheless, TTE has limitations. First, it requires training before being sufficiently skilled to deal with complex cardiac diseases. Second, the precision of the technique must be taken into account, especially when assessing the function during dynamic tests or in emergency situations. Nonetheless, TTE is, without doubt, one of the best imaging tools for bedside diagnosis and evaluation of acute cardiovascular conditions [41, 58].
Bioimpedance and bioreactance analysis
The method of electrical bioimpedance analysis (BIA) is a non-invasive method that uses the different response of tissues to an electric current flow. A schematic application of this method is shown in Figure 1 B. The distribution of fluids in the body can be evaluated by total fluid volume, intracellular fluid, and extracellular fluid. Thus, this method can be used to assess the effects of treatment in patients with decompensated AHF, fluid distribution, and even CO [59]. Although assessment of CO by BIA is possible, its accuracy and reproducibility are controversial. In meta-analyses comparing CO evaluation by BIA and thermodilution as a reference method, the measurement error reached about 40%. Thus, BIA cannot be a reliable method of CO evaluation if accurate measurement is needed. It can probably be used to monitor the trend of CO changes as an evaluation of the effectiveness of the treatment [43]. Of note, BIA results have been shown to be associated with length of hospital stay. The greater the level of fluid state assessed by BIA, the longer the hospital stay of patients with AHF. This suggests that BIA can be used for risk assessment in these patients [60]. The application of a method that uses a flowing electric current may be of concern to people with implanted cardiac devices. However, this procedure has been shown to be safe in such patients and does not affect the batteries or the parameters programmed into the appliances [61].
Bioreactance analysis (BRA) is an improved method based on bioimpedance. BRA analyses changes in the frequency spectrum of the delivered oscillating current [43]. BRA can provide better estimation of CO compared to BIA, and its efficiency is similar to the results obtained by thermodilution. In a direct comparison of BIA and BRA for CO measurement, BIA underestimated the value obtained compared to BRA and thermodilution [62]. In addition, BRA showed CO changes faster than thermodilution. Thus, it appears that CO can be effectively and fully non-invasively monitored using BRA, with a correlation agreement with thermodilution > 0.70 [62]. It was also demonstrated that the BRA method can be successfully used to guide fluid therapy in haemodynamically unstable patients. It allows a quick and effective assessment of whether the patient is responding to fluid therapy in a passive leg-raising test, which is crucial in the initial treatment of these patients [43]. However, there is a lack of studies evaluating the usefulness of BRA in patients with AHF, and current research is based primarily on perioperative care. Hence, more studies are needed to fully recommend this method for haemodynamic monitoring of patients with AHF.
Pulse contour analysis
The pulse contour analysis (PCA) method provides an evaluation of CO based on continuous analysis of the arterial pressure (AP) waveform [44]. The AP waveform is a complex physiological signal consisting of the interplay between stroke volume, vascular compliance, and systemic vascular resistance. There are many algorithms and commercially available devices to assess CO using this method. They can be divided into invasive, minimally invasive, and non-invasive [44]. We mentioned the invasive method earlier. Transpulmonary thermodilution (TPTD) is used to calibrate the information obtained with PCA, making it closer to the actual values and providing a reference method for other measurements. Minimally invasive methods do not need venous catheters for their function and usually do not use external calibration. They use database information and nomograms based on demographic and biometric data for internal calibration [63]. This is also similar for non-invasive methods. These include the use of finger cuffs or sensors placed over the radial artery [64, 65]. They can thus read AP waveform changes and, after analysis and internal calibration, provide an approximate CO score. The usefulness of these methods depends strictly on the clinical situation. The less invasive the method, the more the measurement can differ from the true value. Nevertheless, observing CO trends can be used, for example, to guide fluid therapy. It is possible to assess a patient’s response to fluid therapy during a passive leg raising test [66].
However, many situations can interfere with the correct measurement of CO with PCA. These include age-dependent vascular compliance, increased vascular tone, or undesired sensor movement. In addition, PCA cannot be used in patients without pulsatile blood flow, e.g. those connected to extracorporeal membrane oxygenation (ECMO) or with a LV assist device [44]. Invasive monitoring using PCA should not be used if there are contraindications to intravascular catheter placement.
Oesophageal Doppler
Oesophageal Doppler has been used since the 1990s. It is a type of ultrasound imaging in which a D-shaped Doppler probe is positioned in the oesophagus and emits ultrasound waves (Figure 1 C). Its purpose is to precisely, continuously, and minimally invasively monitor critically ill patients [45]. It calculates CO based on descending aorta’s diameter, blood flow velocity in the aorta, and CO distribution to the descending aorta [67]. The cross-sectional area of the aorta is either estimated based on the patient’s characteristics (such as age, height, and weight) or measured using transoesophageal echocardiography.
Aortic blood flow variations are suggested to be precise predictors of fluid responsiveness in patients on mechanical ventilation [68]. In addition, this technique indirectly provides information concerning the cardiovascular state of the patient (preload, afterload, and contractility). It is suggested that CO measured by oesophageal Doppler, compared with thermodilution, is also reliable [45]. This technique, however, is more suitable for the operation room than for the intensive care unit because the probe can move in the oesophagus in less sedated patients [46]. It is also inserted blindly, which may result in poor positioning and consequently incorrect CO estimation [67]. Moreover, the diameter of the aorta, when estimated, is not constant because it is vulnerable to transmural pressure. Therefore, during shock management, when the mean arterial pressure (MAP) can change rapidly, CO should not be tracked by changes in estimated aorta blood flow [46]. It is contraindicated to insert an oesophageal Doppler probe in patients with previous surgery, stent, carcinoma, or oesophagus varices, in coarctation of the aorta, or when an intra-aortic balloon pump id used, along with coagulopathy [69].
Pulmonary artery catheterization
The pulmonary artery catheter (PAC) (Figure 2 A) was introduced in the 1970s and remained the only option for haemodynamic monitoring for a long period of time. The use of PAC has fallen out of favour in the past 2 decades because of its invasiveness and lack of benefit of its use in critically ill patients [17, 47, 70]. Nevertheless, it has been suggested that PAC might still play a key role in the haemodynamic monitoring of critically ill patients. At present, PAC is recommended in patients with refractory shock associated with RV dysfunction [47, 70]. Observational trials demonstrate that the use of a pulmonary artery catheter is associated with significant modifications in the therapy and that these may be associated with improved outcome [71]. Its advantage is to continuously monitor PAP, right atrial pressure (RAP), pulmonary capillary wedge pressure (PCWP), CVP, and mixed venous oxygen saturation (SvO2) (determinants of adequate oxygen delivery). PAC only provides either intermittent or semi-continuous CO measurements and is unable to reliably track short-term changes in CO. Nonetheless, it is considered a gold standard in CO measurement [47]. PAP measurement is particularly advisable in cases of RV dysfunction, where evaluation of the RV afterload is crucial for diagnosis as well as for guiding therapy [72].
Figure 2
Diagram showing the principle of pulmonary artery catheterization (A) transpulmonary thermodilution (B)
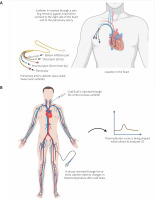
Another parameter that helps to predict severe RV dysfunction is the pulmonary artery pulsatility index (PAPi). The PAPi is used for continuous therapy monitoring, allowing its adjustment [73].
Measurements of PCWP are indicators of possible severe LV failure or severe mitral valve stenosis/regurgitation. By measuring PCWP, the dosage of diuretic drugs and vasodilators used to reduce pulmonary capillary and venous pressure can be adjusted, thereby reducing pulmonary oedema. It is also used to evaluate and diagnose pulmonary arterial hypertension and in differentiating cardiogenic shock (PCWP > 15 mm Hg) from non-cardiogenic shock (PCWP ≤ 15 mm Hg) [28]. PAC is contraindicated in patients with right-sided endocarditis, masses, or tumours, relatively – with severe thrombocytopaenia and coagulopathy. Special care should be taken when inserting the catheter in patients with right-sided valve disease or with left bundle branch block [74]. Differentiation of clinical conditions based on the parameters obtained by PAC in clinical practice are shown in Table III [17].
Table III
Differentiation of clinical conditions based on parameters obtained by pulmonary artery catheter [17]
Transpulmonary thermodilution
TPTD with arterial pulse contour analysis is the most commonly used alternative to PACs. It is a minimally invasive method and requires the placement of an arterial line and central venous access. Through a catheter in a central vein, cooled fluid is injected into the circulation. The bolus passes through the right heart, pulmonary circulation, and LV, and finally reaches the femoral artery. The blood temperature change is detected with a sensor located on an arterial catheter and a thermodilution curve is then being constructed (Figure 2 B) [20]. TPTD itself provides intermittent measurements of CO. However, it can be exploited to calibrate the arterial pulse contour analysis method, which provides continuous measurements of CO, but requires frequent calibration (every 6–8 h). The reproducibility of TPTD for measuring CO has been determined to be 6.1 ±2.0% in adult studies and 3.9 ±2.9% in paediatric studies [75]. These results demonstrate the high reproducibility of TPTD, especially in the paediatric population. However, there is a need for an average of 3 bolus injections to obtain the mean CO value [75]. Using the thermodilution curve, the information about extravascular lung water (EVLW), pulmonary vascular permeability (PVP), global end-diastolic volume (GEDV), and computed cardiac function index (CFI) can be obtained, which are crucial parameters to assess patients with decompensated AHF [17]. Differentiation of clinical conditions based on parameters obtained by transpulmonary thermodilution is shown in Table IV.
Table IV
Differentiation of clinical conditions based on parameters obtained by transpulmonary thermodilution [17]
Performing a TPTD requires close attention. Inappropriate injection volume, irregularity of the injection speed, leakage of the circuit, and inadequate bolus temperature (for most accurate CO estimation it should be less than 8°C) can interfere with correct CO measurement [20]. The main advantage of transpulmonary thermodilution is the ability to measure EVLW and PVPI. These parameters can be very useful in planning appropriate fluid therapy. They can indicate the risk of fluid overload, which is important in patients with AHF [76]. The GEDVI parameter better estimates the preload than using pressure markers of preload. However, it cannot distinguish between LV and RV dysfunction. TPTD allows the calculation of the CFI, which indicates decreased heart contractility [20]. In these situations, performance of TTE is indicated. This will allow additional information to be gained, including an accurate assessment of the EF and possible reasons for its change.
The limitations of TPTD include intermittent CO measurement, requiring the use of an additional technique to obtain a continuous measurement (PCA). In addition, TPTD measurements may be distorted in many clinical situations. For instance, EVLWI and PVPI will be unreliable in the case of pulmonary embolism, large pleural effusions, and after lung resection [20]. Furthermore, TPTD contraindications include intracardiac leaks because they cause significant abnormalities in measured parameters [77].
The main indication for the use of TPTD is AHF with no response to the initial therapy. The risk of invasiveness and costs of this procedure should be considered before its use. In practice TPTD should be combined with echocardiography, which allows comprehensive assessment of haemodynamic status and may identify the cause of AHF. Furthermore, there is insufficient evidence to answer the question of whether TPTD improves outcomes in patients with AHF. It may be that patients do not benefit in the long term from TPTD, as was the case with PACs methods [76]. There is a need for further studies to establish the impact of TPTD for long-term outcomes in AHF patients.
Conclusions
Haemodynamic monitoring is an essential part of the AHF treatment. It provides important information to differentiate the type of dysfunction, cause, and severity. Fluid therapy is a key component of treatment in these patients, and appropriate haemodynamic monitoring allows for optimization of fluid administration. Echocardiography is one of the best methods to evaluate the haemodynamic status. However, in the setting of haemodynamic instability, it should be supported by methods that allow continuous measurement of haemodynamic parameters. Depending on the clinical situation, invasive or non-invasive methods may be used. The accuracy of each method, and advantages and the inconveniences associated with them, should then be considered. Finally, there is a need for more studies comparing the accuracy of different methods in AHF and their impact on the long-term outcomes.