Summary
This study sought to describe bioresorbable vascular scaffold (BVS) ABSORB healing in vein grafts by optical coherence tomography (OCT) and high-definition intravascular imaging (HD-IVUS) at long-term follow-up. Complete scaffold coverage was observed only 48 months after implantation. There was a difference in NIH thickness (1.27 (0.953–1.696)] vs. 0.757 (0.633–0.848), p < 0.001) between a native coronary artery and BVS scaffolds at 48-month follow-up. HD-IVUS presented plaque burden up to 67% at the segment of BVS implantation in the vein graft at 48-month follow-up.
Introduction
In the last years, implantation of bioresorbable vascular scaffolds (BVS) have attracted worldwide interest as an equally valuable alternative to drug-eluting stents to treat coronary vessel disease. The outcomes of the first clinical trials were so promising that BVS implantation became a commonly accepted therapy of coronary artery disease [1, 2]. Bioresorbable vascular scaffolds was not only used to treat simple coronary lesions but was also implanted in bifurcations, chronic total occlusions, in-stent restenosis and de novo lesion of saphenous grafts [3–6]. Unfortunately, the initial enthusiasm vanished because the long patient follow-up resulted in increased incidence of late scaffold thrombosis after BVS implantation [7, 8].
Although BVS implantation is now not recommended during percutaneous coronary interventions [9], its remodeling and healing in a different clinical setting have not been comprehensively described yet [10]. One such unusual clinical scenario is the implantation of BVS to de novo lesions of vein grafts [11, 12].
The first clinical observations presented promising results of BVS implantation in vein grafts, and intravascular imaging presented favorable vein graft healing after BVS implantation at short-term follow-up [5, 13, 14]. However, there were no data presenting a serial assessment of vein graft healing after BVS implantation at long-term follow-up.
Aim
The following study sought to describe BVS healing in vein grafts by optical coherence tomography (OCT) and high-definition intravascular imaging (HD-IVUS) at long-term follow-up. The obtained results were compared with BVS healing in native coronary arteries.
Material and methods
It was a single center study evaluating the vessel healing after BVS ABSORB (Abbott Laboratories, USA) [1] implantation in vein grafts by multimodality imaging including optical coherence imaging (OCT) and high-definition intravascular ultrasound (HD-IVUS) in patients with stable coronary artery disease (CAD) and acute coronary syndromes (ACS). The study was approved by the Ethics Committee of the Medical University of Silesia (KNW/0022/KB1/39/18) and conforms to the Declaration of Helsinki. All of the patients were enrolled in the study only after the patients gave their informed written consent.
Inclusion/exclusion criteria
The study included patients with a history of coronary artery bypass grafting (CABG) utilizing vein grafts with recurrent stable CAD or ACS to implant and to perform serial intravascular imaging of BVS. Furthermore, the study included patients 48 months after BVS implantation in native coronary arteries to compare vessel healing after BVS implantation between SVG and native coronary arteries. The study exclusion criteria were as follows: age < 18 years old, glomerular filtration rate less than 45 ml/min/1.73 m2, severe valve disease warranting redo cardiac surgery and contrast allergy.
Optical coherence tomography imaging
Optical coherence tomography imaging was performed after the scaffold implantation in SVG and at the follow-up. It was also performed 48 months after BVS implantation in native coronary arteries. The St Jude iLumien OPTIS Medical system was used for OCT imaging. The OCT probe (a mid marker of the OCT Dragonfly catheter) was positioned 5 mm distally to the scaffold intended to analyze. All OCT imaging was performed using automated pullback triggered by the manual injection of contrast.
Optical coherence tomography image analysis
CASS intravascular software 2.0 (Pie medical company) was used for offline analysis of the implanted BVS. The region of interest was selected between the proximal and distal edges of the BVS visible by OCT as struts occupying more than 180 degrees of the lumen’s circumference. The analysis was performed every 1 mm to measure lumen area (LA), lumen diameters (LD) and endoluminal and out scaffold area (SA). Endoluminal SA was measured at the inter circumference of polymeric struts and out SA was measured at the outer rim of polymeric struts. The eccentric index (EI) was measured as follows: EI = 1 – (minimal lumen area/maximal lumen area). Lesions with EI > 0.3 were defined as eccentric lesions.
Polymeric struts apposition was also assessed, and if there was a gap between the polymeric strut and the vessel’s lumen contour, malapposition was diagnosed [15]. At the follow-up, polymeric struts’ coverage by neointima was also assessed. The complete coverage of BVS by neointima was identified if four corners of the polymeric strut had lost the right-angle shape with signs of tissue coverage [16]. To measure the tissue thickness the distance from every black box to the lumen contour was measured. Since the thickness of the BVS contours is 30 μm, this value was subtracted from the final tissue measurement to present neointimal hyperplasia (NIH, μm). Neointimal area was measured by subtracting as follows: endoluminal SA – (lumen area + malapposition area).
HD-IVUS image analysis
HD-IVUS imaging was performed using the ACIST HDi system and ACIST Kodama IVUS catheter device. The region of interest was the segment of the artery where the BVS was previously implanted. Quantitative grey-scale IVUS measurements were performed every millimeter in scanned coronary segments. Cross-sectional images were quantified for lumen diameters and area, external elastic membrane (EEM) diameters and area, total plaque area (TPA) and plaque burden (PB). Since all HD-IVUS imaging was performed at 48 months after implantation no BVS strut detection was performed. TPA was calculated as the difference between EEM area, and PB was calculated as total plaque area (TPA) divided by EEM area × 100 (%).
Statistical analysis
Continuous parameters were reported as mean with standard deviation and median with the first and the third quartiles. Discrete data were summarized as frequencies and group percentages. Wilcoxon signed-rank test and the χ2 test with Rao and Scott adjustment were used for comparison of continuous and categorical data, respectively. P-value < 0.05 was considered statistically significant. Analyses for statistical computing were performed using MedCalc version 18.6 (MedCalc Software, Belgium).
Results
Study group
The study group consisted of 6 patients. Forty-eight months imaging was performed in 5 patients. The first one could not have the intravascular imaging at 48-month follow-up because she died 40 months after BVS implantation due to a non-cardiac cause (lymphoma). The others did not experience any adverse cardiac events at 48-month follow-up. All patients received DAPT for the 12 months after BVS implantation. Patients and BVS characteristics, and the study flow chart are presented in Figure 1.
Figure 1
Study chart flow. The study chart flow presents the time of optical coherence tomography (OCT) and high definition intravascular (HD-IVUS) imaging of ABSORB implanted in vein grafts and coronary arteries
HA – hypertension, HL – hyperlipidemia, DM – diabetes mellitus, CABG – coronary artery bypass grafting, GFR – glomerular filtration rate (ml/min/1.73 m2).
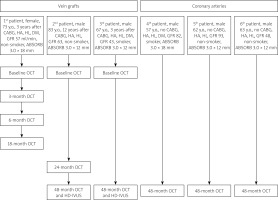
Serial OCT follow-up of BVS after its implantation in SVG
The first BVS was observed at baseline and at 3, 6 and 18 months after implantation. The MLA and MLD decreased after 3 months, and then it significantly increased at 18 months. There were no differences in NIH and the neointimal area between each time point respectively. Interestingly, endoluminal SA was systematically increasing at each time point, but the out SA increased only in the sixth month after BVS implantation (Figure 1, Table I). The second BVS was observed at baseline, 24 and 48 months after its implantation. MLA and MLD decreased at 24- and 48-month follow-up as compared to baseline values (Table I). There were no differences in NIH and the neointimal area between each time point. The endoluminal SA and out SA increased over the time of observation (Figure 2, Table I).
Table I
Serial OCT assessment of ABSORB scaffold implanted in SVG
Figure 2
Representative serial OCT images of ABSORB implanted in vein graft. The figure presents OCT images of two bioresorbable vascular scaffolds (ABSORB) implanted in vein grafts at baseline, 3-, 6-, 18-, 24- and 40-month follow-up. The figure presents the measurements of neointimal thickness for every scaffolds strut. The two blue triangles present uncovered struts of ABSORB at 3-month follow-up
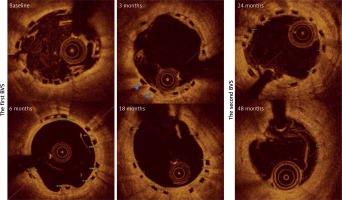
48-month follow-up after BVS implantation in SVG
There were 26 OCT cross-sections analyzed at baseline and 17 OCT cross-sections analyzed at 48-month follow-up for both BVS. Out of 202 scaffold struts, there were only 67 (33%) black boxes visible at 48-month follow-up. The MLA (mm2, 7.45 (4.65–8.75) vs. 4 (3.51–3.01), p < 0.001) and MLD (2.81 (2.25–3.05) vs. 1.91 (1.81–2.21), p < 0.001) were smaller at 48-month follow-up as compared to baseline. The endoluminal SA (7.3 (4.85–7.9) vs. 5.5 (5.2–5.7) p = 0.464) and out SA (9.35 (6.37–10.25) vs. 7.16 (6.86– 7.83), p = 0.691) decreased at 48-month follow-up. HD-IVUS was not able to visualize struts at 48-month follow-up and presented MLA = 3.4 mm2, MLD = 2 mm, PB = 44% and TPA = 2.7 mm2 and TPV = 33.7 m2 for the first patient, and MLA = 4.6 mm2, MLD = 2.3 mm, PB = 62% with TPA = 6.2 mm2 and TPV = 68.7 mm3 for the second patient. HD-IVUS also presented the echogenic rim of neointima remaining after the BVS implantation (Figure 3).
Figure 3
Representative angiography, OCT and HD-IVUS imaging of ABSORB implanted in the vein graft at 48-month follow-up. A – The coronary angiography presents the vein graft 48 months after ABSORB implantation. The dashed lines indicate (1, 2, 3) the position of HD-IVUS (B, D, F) and OCT cross-section images (C, E, G). The white arrows indicate the echogenic rim of the remaining neointima (B, D, F) and blue triangles indicate black boxes remaining (C, E, G) after ABSORB implantation
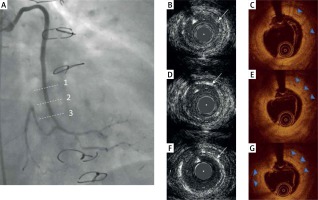
48 months after BVS implantation in SVG vs. native coronary artery
There were 41 OCT cross-sections and 268 struts analyzed in native coronary arteries and 17 cross-section and 67 struts analyzed in SVG. There were no differences in MLA (4.8 (3.6–8.9) vs. 4.0 (3.5–5), p = 0.228), endoluminal SA (6.15 (5.1–11.1) vs. 5.5 (5.2–5.7), p = 0.1048) and out SA (7.72 (6.61–13.38) vs. 7.16 (6.87–7.83), p = 0.3664) between native and SVG scaffolds. However, there was a difference in NIH thickness (1.27 (0.953–1.696) vs. 0.757 (0.633–0.848), p < 0.001) between a native coronary artery and BVS scaffolds at 4 years follow-up.
Discussion
It is the first description of BVS healing by serial OCT imaging at long-term follow-up. The main finding of the study was as follows: 1) BVS healing in vein grafts was a dynamic process with the reduction of lumen area during the first 6 months, subsequent lumen growing up to 18 months and final lumen reduction observed at 24- and 48-month follow-up. The observed lumen remodeling was not accompanied by increasing neointimal hyperplasia. 2) OCT imaging presented 33% of remaining scaffold black boxes, and HD-IVUS imaging revealed a significant plaque burden and neointimal rim at the site of BVS implantation at 48-month follow-up. 3) The vessel remodeling that occurred after BVS implantation was similar in vein grafts and native coronary arteries at 48-month follow-up. 4) The scaffold coverage was not complete in vein grafts until the 48th month after implantation.
The previous data from native coronary arteries indicated that neointimal hyperplasia was observed during the first 24 months after the BVS implantation. It was observed in stable coronary patients as well as in those with ACS. For both stable CAD and acute coronary patients, the fast NIH was observed in the first year after implantation, and then it slowed down. The neointima was ~600 μm thick at 12 months after BVS implantation in stable CAD patients [17], and ~300 μm or ~430 μm in STEMI patients at 12- and 24-month follow-up respectively [18]. The observed BVS healing in the vein graft showed that the thickness of neointima (~600 μm) at 24-month follow-up was similar to that observed in BVS implanted in patients with stable CAD at 24-month follow-up and was twice as thick as in patients with STEMI [17, 18]. There was also no significant increase in NIH after 3 months of BVS implantation in the vein graft, suggesting its faster healing.
In our study, we observed a nearly complete scaffold coverage at 3-month follow-up, which is in line with previous reports [13]. Long-term observation showed a stable thickness of BVS coverage in the vein graft, but full scaffold coverage was observed only at 48-month follow-up. It is in contrast with data obtained from BVS implanted in native coronary arteries [19]. Almost complete (~99%) coverage was found after BVS implantation in stable CAD and STEMI patients at 12-month follow-up [17, 18].
Interestingly, the vein graft lumen presented dynamic remodeling after BVS implantation, which is not in line with previous reports. The BVS lumen area remained stable after 12 months in both patients with stable CAD and ACS [18, 20]. Previous studies also showed constant remodeling of the vessel eccentricity in the first 24 months after BVS implantation in SCAD [10, 21]. However, a higher percentage of eccentric lesions was observed after the index procedure, within a time the vessel concentricity increased and remained stable after 2 years. Our study showed that BVS characterized stable scaffold concentricity up to 48 months after the implantation. It may be explained by the fact that vein graft lesions are less calcified and thus an appropriate scaffold shape was much more easily achieved as compared to native coronary arteries [22, 23]. Additionally, the stable BVS shape may explain the lack of malapposition during the follow-up, which in line with the animal model observations [24], but in contrast to human studies. BVS malapposition was observed even up to 36 months after the implantation [20]. Interestingly, HD-IVUS presented an echogenic rim at 48 months after implantation. Since the OCT images did not present calcifications, the rim represents the acoustic tissue properties at the site of BVS implantation. Further studies are warranted to determine what is left in the vessel wall after BVS absorption.
This is the first report presenting the 48-month results of BVS implantation in vein grafts in comparison to native coronary arteries. It showed that NIH analyzed on the remaining scaffold was higher as compared to that observed in native coronary arteries. This, together with incomplete scaffold strut coverage, suggests that the BVS is less favorable to vessel healing in vein grafts. Greater NIH may be promoted by the lower shear stress in the SVG, as compared to native coronary arteries [25]. Furthermore, IVUS data revealed hypoechogenic lesions at the site of BVS implantation at 48-month follow-up, but their burden did not differ from those observes 3 years after BVS implantation in native coronary arteries [20]. The previous report documented progression of calcification at the segment of previous BVS implantation [26].
Study limitations
The study enrolled a small number of patients, which is the main limitation. The serial intravascular imaging assessment was performed only in 2 patients, which makes it hard to draw any general conclusion from these observations. Unfortunately, it was impossible to enroll more patients because the distribution of ABSORB was stopped during the study period. The results come merely from the cross-sectional analysis. There was no propensity matching between patients with BVS implanted in vein grafts and native coronary arteries, which could also bias the comparison of vessel healing between these two clinical scenarios.
Conclusions
The serial intravascular imaging of BVS implanted in the SVG presented dynamic lumen remodeling but with stable scaffold concentric shape. The neointimal hyperplasia was not excessive, but the scaffold coverage was not complete even 24 months after the implantation. HD-IVUS presented a remaining plaque at the site of BVS implantation at 48-month follow-up.