Summary
Transcoronary delivery of stem cells is understood to be the most physiological way of delivering therapeutic cells to the infarcted myocardium. However, current delivery techniques are suboptimal, as the proportions of delivered cells are small (typically ~5%) and the delivery mode (such as the “stop-flow”, coronary-occlusive technique) produces myocardial ischemia and arrhythmias. The new CIRCULATE catheter, due to its unique design, delivers cells under normal coronary flow, improving the distribution inside the vessel with laminar flow (external “layers”), enabling physiologic adhesion and diapedesis of the therapeutic cells. We show that trans-coronary delivery of neonatal stem cells using the CIRCULATE catheter in a pig model of acute myocardial infarction is safe and is associated with an effective reduction of the infarct size.
Introduction
Cardiac progenitor cells (CPCs) are extensively described in the literature. They are mesenchymal stem cells derived from the right atrial appendage and are characterized by high expression of receptor tyrosine kinase (C-kit+) [1]. At the same time, they do not possess CD45, Lin and tryptase. Animal models of acute and chronic ischemia showed the efficacy of CPCs in ameliorating left ventricle (LV) dysfunction [1]. Studies characterizing CPCs from samples from young patients undergoing cardiac surgery procedures showed that the density of CPCs found in the myocardium decreased with age; in neonates (age < 30 days) the density was 9% compared to 3% in older children [2–4]. The number of CPCs in other parts of the heart is very low [4]. Both age and different environmental conditions (hypoxia) can impact secretome production, and by doing so, they influence CPCs’ paracrine capabilities [5]. Sharma et al. observed that neonatal CPCs (nCPCs) had better regenerative potential in comparison to adult CPCs in the myocardial infarct (MI) rat model [5]. Additionally, CPCs reduced inflammation in the infarct border zone and fibrosis [5]. Those findings are consistent with another animal model explaining the role of CPCs in a right pressure overload model. The use of nCPCs improved the right ventricular function and preserved it until 4 weeks. In contrast, adult CPCs (aCPCs) coming from older donors did not present such exquisite capabilities [5]. Another interesting finding came from Agarwal et al., claiming that hypoxia improved efficacy of pediatric human CPC-derived exosomes in a rodent MI model [6]. Neonatal hypoxia conditioned CPCs may have the most promise in the field of stem cell therapy [7].
The CIRCULATE catheter is designed to enable physiologic intracoronary delivery of cell-based products, including therapeutic cells.
Aim
The first purpose of this study was to investigate, in a large-animal model of acute myocardial infarction, the safety and efficacy of the novel catheter for intracoronary infusion of stem cell therapy. The second aim was to present the results of neonatal mesenchymal stem cell (nMSC) and total conditioned medium (TCM) based therapies, delivered through the coronaries in the same setting.
Material and methods
The CIRCULATE catheter for transcoronary delivery of cell-based therapies is a rapid-exchange infusion-perfusion system with several unique features. The system includes an expandable intracoronary reservoir (housing the cell/cell product(s)/drug(s) suspension) that possesses spirally placed side holes of varying diameter, enabling controlled perfusion delivery of the investigational medical/therapeutic product(s). The crossing profile of the system is 2.2 F/0.7 mm (CIRCULATE Catheter, Protected Design No 72837, Patent Office of the Republic of Poland). It has a monorail design, but the balloon is fenestrated to allow slow infusion of the injected material down the side walls of the balloon (oozing), in a spherical formation, instead of jetting from the front end of the balloon at a higher speed and being washed away from the target area. This unique method of infusion enables physiological (rather than stop-flow) transcoronary delivery of therapeutic cells, allowing longer contact between the infused cell product and the endothelial surface. This may allow more cell or cell product retention within the targeted treatment area, the myocardium.
Cell culture and cell derived products were prepared and used as previously described [2, 3, 5].
Femoral cannulation
We used a closed chest model to create MI in female Yorkshire pigs (20 ±2 kg). All the pigs underwent general anesthesia. Baseline transthoracic echocardiogram (TTE) and blood collection were performed. Femoral arterial access was obtained percutaneously, using ultrasound guidance, via the modified Seldinger technique (or cutdown when necessary). A 5 French arterial vascular access device was placed, then changed over the wire to an 8 French introducer. The arterial lines were secured to the skin for the duration of the operation using silk or nylon suture. Heparin 100–300 units/kg was administered intravenously (IV) and activated clotting time (ACT) measured no sooner than 3 min later, with the goal ACT of 200–250 s. ACT was measured every hour while the introducer sheath was in place, and an additional 100–300 units/kg of heparin were administered IV as needed to maintain the ACT goal.
Induction of myocardial infarction
All procedures were performed by a single surgeon. Amiodarone 3 mg/kg and lidocaine 1 mg/kg infusions were started prior to induction of myocardial infarction. Internal mammary artery (IMA) guide catheters were used to gain access to the left main coronary ostium, perform coronary angiography, and deploy an intracoronary angioplasty balloon. The catheter was advanced into the aortic root over a guide wire under fluoroscopy. Coronary angiography was conducted with iodinated contrast medium (iohexol, Omnipaque) administered into the left coronary artery. A 0.014” guidewire was advanced into the left anterior descending artery (LAD), and a 2.5 mm angioplasty balloon was advanced to the distal third of the LAD and inflated to the nominal pressure (8 atm). Repeated angiography was done while the balloon was inflated to ensure complete occlusion and assess for any complications. The balloon was left in place for 60 min. Monitoring continued as described above during this time. After 60 min, the balloon was deflated gradually and removed. Reperfusion was allowed for 15 min, and post-MI echocardiography was performed. The CIRCULATE catheter was then introduced over the wire and nMSC, TCM or a combination of both was administered into the LAD. The pig was allowed to recover and monitored for 48 h.
Euthanasia
Before euthanasia the final echocardiogram was performed. The LAD was accessed with the guide catheters and the balloon was placed exactly in the same position as during the first procedure. After inflation of the balloon, 40 ml of 1.8% gentian violet was administered IV. At the same time, pigs received concentrated potassium chloride (KCl) solution to arrest the heart. After euthanasia the heart was harvested through the right anterolateral thoracotomy.
Staining and measurements
Infarct size (IS) and area at risk (AAR) are crucial parameters in all research investigating IS limitation [8]. The AAR represents the entire myocardial perfusion bed distal to an occluded coronary artery and determines the final IS area [9, 10]. During an acute myocardial event, the myocardium in the AAR will progress to necrosis beginning with the central subendocardial tissue and spreading through the epicardium and toward the periphery of the AAR [11]. Following occlusion of a coronary artery, the perfused myocardium turned violet (the effect of 1.8% gentian violet) and the AAR was left unstained [12]. The left ventricle was dissected from the rest of the heart and sliced transversally at a thickness of 8–10 mm and immersed in 2% TTC (2,3,5-triphenyltetrazolium) solution for 20 min at 37°C. The viable myocardium was stained as the water-soluble compound TTC was converted by active mitochondrial dehydrogenases into an insoluble red precipitate. The extent of staining correlated with the number of viable mitochondria and differentiated viable and nonviable tissue [13]. The infarcted tissue remained unstained and appeared whitish. Each slice was weighed before and after TTC staining and the results were recorded. Photographs were taken before and after TTC staining from both sides of all slices. IS and AAR were calculated using an area-based method and Image J software. The area of the infarcted region and/or AAR was expressed as a percentage of the total area of that slice. Afterwards, IS, AAR and LV mass were calculated. The IS/AAR, IS/LV and AAR/LV ratios were obtained. For rat and swine heart this method is sufficient because these species’ hearts do not have many collaterals which could deliver the blood to the occluded area [14].
Troponin was assessed from blood samples taken at baseline and after 48 h. The blood was centrifugated and frozen at – 80°C and the plasma was sent to the external laboratory for the assessment of troponin and expressed in ng/ml.
Transthoracic echocardiography was performed by a single echocardiographer using a General Electric Vivid Q Ultrasound and 3S probe (General Electric, New York, NY). Using parasternal short axis view the and M mode the ejection fraction was calculated. All images were reviewed and analyzed by a single blinded investigator.
Statistical analysis
Data were analyzed using GraphPad Prism software. When comparing 2 conditions, we used Student’s t-test with the Mann-Whitney U test where appropriate. More than 2 comparisons were made using one-way analysis of variance (ANOVA) followed by Dunn’s or Tukey’s post hoc test. Two-way ANOVA with Bonferroni correction was used for grouped analysis of echocardiographic data. Probability values < 0.05 were considered significant.
Results
From March 2019 to January 2021, 39 female pigs underwent the procedure. Different types of stem cell therapies were administered intracoronarily (Table I).
Table I
Mortality in acute swine myocardial infarction model with intracoronary delivery of neonatal mesenchymal stem cell therapy with CIRCULATE catheter
No. | Treatment | No. of treated pigs | Deaths |
---|---|---|---|
1 | Placebo | 7 | 2 |
2 | 10 M nMSC | 9 | 0 |
3 | 20 M nMSC | 4 | 0 |
4 | Combo 10 M nMSC + 2 mg TCM | 6 | 0 |
5 | Combo 20 M nMSC + 2 mg | 4 | 0 |
6 | TCM 2 mg | 6 | 0 |
7 | TCM 5 mg | 3 | 0 |
Two pigs died due to malignant arrhythmia during the time of balloon inflation despite the administration of antiarrhythmic drugs. The overall mortality was 6.3%.
The CIRCULATE catheter provided 100% success in delivery of stem cells and TCM to the target vessel. None of the animals demonstrated perforation of the heart, dissection of the aortic root or coronary ostia. All used catheters were examined for potential damage, laceration or kinking and showed no insult.
As expected, the gross inspection of the heart revealed the presence of fresh scarring in the anteroseptal and anterior LV wall (Figure 1). The IS to AAR ratio was calculated for all treated groups (Figure 2). A significant difference in IS/AAR was observed in the high dose nMSC treated group as well as the nMSC 10 M + TCM 2 mg treated pigs versus placebo. Combination of those two treatment options (10 M cells + 2 mg TCM) showed a significant reduction in IS/AAR ratio compared to cell therapy alone. The exclusive use of either dose of TCM had no effect on the infarct size in our study groups (Figure 2 A). There were no significant differences in AAR/LV among all groups, which means our myocardial model was consistent in all the study population (Figure 2 B).
Figure 1
Representation of TTC heart muscle staining. Hearts were treated with placebo (A), 10 M nMSC (B), 20 M nMSC (C), 10 M nMSC + 2 mg TCM (D), 2 mg TCM (E), 20 M nMSC + 2 mg TCM (F), 5 mg TCM (G)
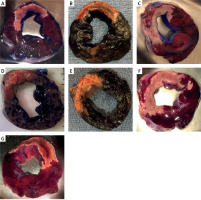
Figure 2
A – Representation of IS/AAR, after different types of intracoronary stem cell therapy in acute MI swine model. B – Representation of AAR/LV, after different types of intracoronary stem cell therapy in acute MI swine model. C – Representation of IS/LV after different types of intracoronary stem cell therapy in acute MI swine model
*,**,***,****significant differences, p < 0.05.
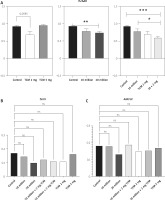
Treated and placebo groups were comparable as far as cardiac function is concerned both at baseline and after the infarct creation (Figure 3 A). We found a significant difference in EF after 48 h between stem cell therapy and placebo groups (Figure 3 B).
Figure 3
Representation of ejection fraction (EF%) values after ischemic-reperfusion injury in acute myocardial infarction swine model: A – after the procedure, B – after 48 h
*,**,***,****significant differences, p < 0.05.
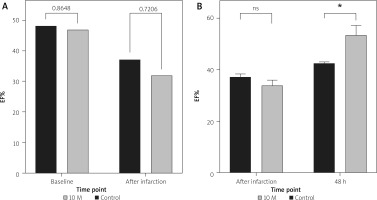
A significant reduction of troponin after 48 h was observed in the treated group in comparison to the placebo (Figure 4), indicating that intracoronary administration of nMSCs is associated with less extensive myocardial infarction.
Discussion
Intracoronary delivery of stem cell is the most common way of delivery of stem cells and their products to the heart. It is a safe and very well-tolerated approach [15, 16]. It can be used during acute infarct and intracoronary procedures [17]. A number of large animal studies have proved this approach to be effective [18].
This paper reports the first use of the CIRCULATE catheter in intracoronary neonatal mesenchymal stem cell therapy injections in a large-animal model. In previous studies there were concerns regarding the safety of intracoronary delivery in chronic settings, especially with the stop-flow technique, which may enhance cell washout and provoke arrhythmias [19], and with high doses of cells which could potentially obstruct the vessel [17]. We believe that the new catheter can resolve that issue, and due to its unique design, the cells can be delivered under maximal preservation of physiologic flow in the coronary vessel, and be better distributed to enable adhesion to the ischemia-activated endothelium and trans-migration to the infarct zone.
Intracoronary injection achieves more homogeneous cell distribution than intramyocardial injection [18, 20]. It has been shown to be safe and efficacious in management of ischemic and non-ischemic heart diseases [18, 20, 21]. However, targeting specific areas of the ischemic myocardium and controlling the rate of infusion could be challenging. Also, under certain conditions, intracoronary injection can be complicated by cell aggregation and microthrombi formation [17], with subsequent myocardial necrosis and infarction. Vulliet et al. observed dose-dependent ST elevation with increased serum troponin at 18 and 22 h after injection, confirming the presence of myocardial necrosis, despite the absence of aggregation before injection and in residual non-injected cells [22].
Intracoronary arterial injection is the clinically preferred method for cell delivery, and it can be done at the time of percutaneous coronary intervention in cases of acute MI [15, 16, 23]. It has proven to be safe and feasible even in children with congenital heart diseases [24]. Cell infusion can be done with either the occlusion technique, where an over-the-wire balloon is inflated and the injection is done through the tip, or non-occlusive injection at a controllable rate [23].
During the occlusion technique, the operator must deflate the balloon to restore the blood flow every 2–3 min to avoid iatrogenic myocardial ischemia, a technique that might induce additional injury. Moreover, special consideration about the balloon size and inflation pressure should be carefully considered [8]. Balloon positioning in relation to the implanted stent is mandatory [8] and might limit the success of targeting the injection to the proximal parts of the ischemic myocardium, as the injection is delivered from the tip of the catheter.
On the other hand, targeting the injection to the ischemic areas and controlling the infusion rate become more challenging in the non-occlusive technique of cell delivery.
A significant reduction in infarct size after using male swine mesenchymal stem cells was reported by Amado et al. They stained the slices with TTC and in the next step the infarct size was confirmed in MRI scans [25]. Similarly to our echo findings, Bolli et al. in a chronic intracoronary swine model observed that in the resident cardiac stem cell treated group the EF increased significantly over the untreated group [26]. Unlike us, the same group did not observe differences in troponin levels among their study groups [26]. Ishigami et al. in their report of the PERSEUS Trial (Cardiac progenitor Cell Infusion to Treat Univentricular Heart) described transient cardiac troponin I and T release, which was fully resolved after 3 days [27]. In our study the troponin levels after 48 h were significantly lower in treated animals, which can be explained by the beneficial influence of stem cells reducing the amount of infarcted tissue.
The neonatal origin of the mesenchymal stem cells we used for the study and the unique design of the CIRCULATE catheter are what distinguish our experiment from others. Our few years’ experience in working with these specific types of cells helped to prove their unique regenerative potential in comparison to the cells derived from adult patients. The same applies to their secretome, which includes more growth factors and different capabilities which come directly from the paracrine activity [5, 6]. The exact mechanisms of nMSC action are being investigated and will be the subject of further publications.