Sepsis is defined as life-threatening organ dysfunction caused by a dysregulated inflammatory response of the host secondary to infection [1]. Septic shock is characterized by circulatory and metabolic/cellular dysfunction and a high risk of death [2]. Together, sepsis and septic shock are among the major causes of health problems worldwide, affecting millions of people annually and with a mortality rate greater than 25% [3, 4].
Haemodynamic support in septic shock includes fluids, vasopressors, and inotropes. Currently, the most highly recommended vasopressors are norepinephrine and vasopressin, while the most recommended inotropic agent is dobutamine [5].
Some patients in shock exhibit dysfunction of the gastrointestinal tract, which is associated with a significant increase in mortality [6–8]. During septic shock, damage to the gastrointestinal tract may occur due both to the direct effect of toxins and inflammatory factors and to ischaemia, with the latter happening when splanchnic hypoperfusion occurs [9]. Sepsis is one of the main phenomena responsible for enterocyte damage or dysfunction due to the following mechanisms: reduced proli-feration of the crypts, reduced enterocyte migration rate along the crypt-villus axis, and increased apoptosis in crypts and villi [10]. Citrulline and plasma or urinary intestinal fatty acid-binding protein (I-FABP) have been used as biomarkers of enterocyte dysfunction and injury [10].
There is evidence that catecholamines have heterogeneous effects on mesenteric blood flow and on the tunica mucosa of the small intestine and may be associated with intestinal mucosal damage [11]. However, some studies [12–14] have shown that dobutamine can counterbalance this deleterious effect of vasopressors by improving intestinal perfusion.
Decreases in serum citrulline concentrations have been reported as a marker of loss of enterocyte mass, intestinal dysfunction and mucosal barrier damage [15]. Piton et al. [16] found that citrulline concentration < 10 μmol L–1 was associated with increased mortality. I-FABP is a potential biomarker of loss of enterocyte integrity, i.e., of ischaemic damage to enterocytes, and reflects the extent of intestinal epithelial ischaemia/damage [10].
Experimental studies have demonstrated that gut mucosal injury can occur as early as 4 h after shock induced by lipopolysaccharide (LPS) administration and despite adequate tissue oxygenation [17–19]. In a pig model of peritoneal sepsis, norepinephrine and epinephrine appeared to divert blood flow away from the mesenteric circulation and decrease microcirculatory blood flow in the jejunal mucosa and pancreas [17, 20]. Therefore, our main hypothesis is that vasopressors may induce gut injury and that the addition of an inodilator, dobutamine, could have a protective effect.
We aimed to evaluate the response of gut biomarkers and superior mesenteric blood flow to different vasopressors with and without dobutamine during the early phase of endotoxic shock in rabbits.
METHODS
Ethics
The protocol of this study was approved by the Ethics Committee on Animal Use (CEUA da Faculdade de Medicina de São José do Rio Preta), approval ID: FAMERP 001-001313/2014.
Study design
Thirty New Zealand rabbits (2.5–3.4 kg body weight) were handled according to the rules of the local Animal Care Committee after institutional approval for animal investigations was obtained. The animals were kept in a vivarium after arrival. The number of animals was arbitrarily defined. The animals were randomly allocated to 5 groups with 6 animals in each group (Figure 1):
Experimental procedures
The animal model was described in detail previously [18]. An initial intravenous bolus of 20 mL of 0.9% saline solution was administered after the induction of anaesthesia. All animals received intravenous lactate Ringer solution at a rate of 2 mL kg–1 h–1 as maintenance fluids until the end of the surgical procedure and a second bolus of 10 mL of 0.9% saline at the end of the surgical instrumentation. Briefly, a midline laparotomy was performed, and ultrasound flow probes (Transonic System Inc., Ithaca, NY, USA) were placed around the abdominal aorta flow (Qao) and superior mesenteric artery (QSMA) immediately below the origin of the celiac trunk for continuous measurement of blood flow. The body temperature, measured by an oesophageal thermometer, was maintained between 36°C and 38°C with an incandescent lamp. The animals could stabilize for 30 to 40 min before starting the experimental protocol. The experimental models were ventilated with the Inter Ventilator (Intermed, Cotia, Brazil).
Experimental protocol
After the stabilization periods, the animals received either placebo in group A (2 mL of 0.9% saline solution) or 1 mg kg–1 LPS (E. coli-055: B5, Sigma-Aldrich) in groups B, C, D and E imme-diately after baseline measurements. LPS was diluted in normal saline (1 mg mL–1) and administered intravenously over 3 min. All groups received continuous intravenous infusion of lactate Ringer over a period of 4 h as maintenance fluids. Fluid replacement in the sham group was due to probable loss of liquid from the opening of the abdominal cavity, thus avoiding possible haemodynamic instability in this group. During this period, the goal of therapy was to maintain MAP greater than 60 mmHg. The blood pressure was measured through cannulation of the right internal carotid artery with a 22G catheter connected to a pressure transducer. If MAP decreased, fluid responsiveness was assessed by administering fluid challenges with 4 to 6 mL kg–1 of 0.9% saline solution and assessing the variation in aortic blood flow. If the variation was higher than 15%, an additional fluid challenge was performed; if lower than 15%, the doses of vasopressor were gradually increased. Norepine-phrine and vasopressin were used at starting doses of 1 μg kg–1 min–1 and 0.01 IU min–1, respectively, and if necessary, they were increased to maintain the MAP. Norepinephrine was used at doses ranging from 0.05 to 2.0 μg kg–1 min–1, and vasopressin was used at doses from 0.01 to 0.04 IU min–1. Dobutamine was combined with the vasopressor in groups C and E at doses of 2.5 to 5.0 μg kg–1 min–1. Mean arterial pressure, temperature, central venous pressure (CVP), Qao, QSMA, arterial blood gas, ScvO2, and serum arterial lactate were measured at baseline and every hour for 4 h. Serum arterial lactate was analysed at the same time of collection, without freezing for storage and later analysis.
As explained above, the objective was to maintain MAP greater than or equal to 60 mmHg; however, it was necessary to maintain minimal doses of vasopressors in respective study groups, even when the MAP exceeded 60 mmHg, to study the drug’s effect. In the sham group if the fluid challenge was not sufficient, no further intervention was applied.
Analytical methods
Analyses of blood gases and serum lactate were performed using an Abbott I-Stat Analyzer. Blood samples were collected for measurement of aspartate aminotransferase (AST), alanine aminotransferase (ALT) at baseline and at the end of the 4-h measurement period and serum citrulline and I-FABP (My BioSource, MBS737587) just at the end of the 4-h measurement period. The samples were centrifuged for 15 min at 3000 rpm and stored in a freezer at –80°C. The measurements of citrulline and intestinal fatty acid binding protein (I-FABP) biomarkers were performed using the ELISA technique with the kits for measuring citrulline (My Biosource; MBS737587) and for I-FABP (My Biosource; MBS2600627). Due to the lack of information from reference data for rabbits, we consider the value of the sham group as a reference of normality.
Grading of tissue oedema and damage
At the end of the experiment, each animal received a lethal injection of xylazine, and samples from the terminal ileum, kidney, liver, and lung were harvested immediately, weighed (wet weight – Ww) and heated at 60°C in a gravity convection oven for 48 h and reweighed (dry weight – Wd) for calculation of the moisture rate (M) by the wet-to-dry weight (W) ratios of each tissue as
Statistical analysis
The data were collected and recorded in an electronic database and subsequently analysed using the statistical software SPSS 25 (IBM Corp., Armonk, NY) and MedCalc 18.11.0. Initially, the characteristics of the models included in the study were described. Experimental models that received vasoactive drugs were compared to the model without the use of vasoactive drugs. The characteristics of each model were evaluated. Normally distributed variables were tested by analysing the histograms using skewness and kurtosis values and by the Shapiro-Wilk test. Parametric continuous data are expressed as the mean and standard deviation (mean ± SD), and nonparametric data are expressed as the median and interquartile range [median (IQR)]; the data were statistically analysed according to the pattern of distribution of the variables. Qualitative data are presented as absolute frequencies and percentages and were compared using Fisher’s exact test. Changes in the parameters as a function of time in each group were analysed by nonparametric two-way ANOVA with Bonferroni correction and by the Kruskal-Wallis test.
For variables with multiple measurements related to haemodynamic variations and blood flow analyses, a general linear model (GLM) was developed to determine the interactions and their effects. This analysis tested the interaction effects among groups and outcomes over time. Thus, repeated measures analysis of variance (ANOVA) was used when the same parameter was measured under different conditions on the same subjects. If the P-value for “groups” is low (P < 0.05), it can be concluded that there is a significant difference between groups. The consistency of the model was tested using Mauchly’s test of sphericity. The final P-value was confirmed by multivariate analysis of variance (MANOVA), and when the interactions were statistically significant, post hoc Bonferroni correction was applied. Bonferroni’s correction compensates for the increased number of hypotheses by testing each individual hypothesis at a higher significance level.
All tests were two-tailed, and a P-value below 0.05 was considered significant. For multiple comparisons, the results were considered significant when the P-value remained below 0.05 even after being multiplied by 5.
RESULTS
MAP was maintained at levels ≥ 60 mmHg in groups B, C, D and E and was significantly higher in group E than in group A (sham) at 2 h (difference of 23.5 mmHg, 95% CI: 3.8–43.2 mmHg; P < 0.01) and 3 h (difference of 29.5 mmHg, 95% CI: 9.8–49.2 mmHg; P < 0.001) (Figure 2).
FIGURE 2
Mean arterial pressure (MAP) in the sham, B – norepinephrine, C – norepinephrine + dobutamine, D –vasopressin and E – vasopressin + dobutamine groups at 0, 1, 2, 3 and 4 h; values plotted are the mean ± standard error
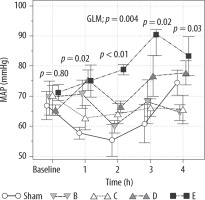
The animal characteristics, amount of fluids given and CVP values were not significantly different relative to the baseline period or among groups (Table 1).
TABLE 1
Perioperative model characteristics
Qao, a surrogate for cardiac output (CO), decreased significantly in group D compared to baseline and was significantly lower than in group B at 3 h and 4 h (difference –35.9; 95% CI: 66.03 to –5.76 mL min–1; P < 0.01). It also decreased significantly in group E in comparison to group B at 1 h (–32.7 mL min–1; 95% CI: –62.8 to –2.56 mL min–1; P < 0.05), at 2 h (–35.7 mL min–1; 95% CI: –65.8 to –5.56 mL min–1; P < 0.01), and at 3 h (–32.5 mL min–1; 95% CI: –62.6 to –2.36 mL min–1; P < 0.05) (Table 2, Figure 3).
TABLE 2
Variation in time of MAP, Qao, QSMA and lactate in the groups at baseline and 1, 2, 3, and 4 h
FIGURE 3
Variation in Qao in the sham, B – norepinephrine, C – norepinephrine + dobutamine, D – vasopressin and E – vasopressin + dobutamine groups at baseline and 1, 2, 3, and 4 h; values plotted are the mean ± standard error
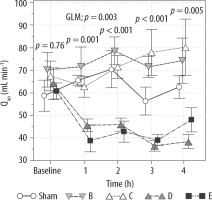
Superior mesenteric artery blood flow was lower in group A than in the other groups. Moreover, it significantly decreased in groups D and E and remained lower in these groups throughout the 4-h experiment (Table 2, Figure 4).
FIGURE 4
Variation in QSMA in the sham, B – norepinephrine, C – norepinephrine + dobutamine, D – vasopressin and E – vasopressin + dobutamine groups at baseline and 1, 2, 3 and 4 h. Values are expressed as the mean ± standard error
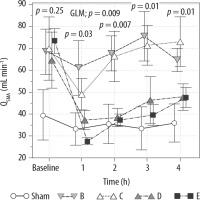
Serum lactate measurements remained constant in group A but increased significantly in the other groups. The greatest variations relative to baseline were observed in groups D and E compared to the other groups, with statistical significance in group D (2.0 ± 0.4 mmol L–1 to 4.6 ± 0.7 mmol L–1; P = 0.002) (Figure 5). ScvO2 fell more sharply in groups D (baseline: 70% ± 55%, 2 h: 55% ± 11%; mean difference 22%, 95% CI: 0.53–44%; P = 0.042) and E (baseline: 79% ± 17%, 2 h: 63% ± 17%; mean difference 24%, 95% CI: 2.3–46%; P = 0.022).
FIGURE 5
Variation in serum lactate levels in the sham, B – norepinephrine, C – norepinephrine + dobutamine, D – vasopressin and E – vasopressin + dobutamine groups at baseline and 1, 2, 3 and 4 h. Values are expressed as the mean ± standard error
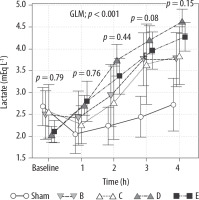
Serum citrulline measurements at the end of the experiment (T = 4 h) were compared among the study groups. Serum citrulline levels were significantly lower in groups D (median 22.1 [10.7–38.8] ng mL–1) and E (median 19.6 [16.3–23.1] ng mL–1) than in group A (median 58.9 [39.1–94.0] ng mL–1) (P = 0.015; corrected to 5 hypotheses) (Figure 6). Serum measurements of I-FABP were compared, and in all groups were statistically significantly different, as compared the sham group (P = 0.03; corrected to 5 hypotheses); group A median 0.49 (0.34–0.6), group B 0.27 (0.26–0.31), group C 0.25 (0.23–0.26), group D 0.25 (0.24–0.28) and group E 0.27 (0.26–0.56) (Figure 6).
FIGURE 6
Serum citrulline and I-FABP measurements in the sham, B – norepinephrine, C – norepinephrine + dobutamine, D – vasopressin and E – vasopressin + dobutamine groups at the end of 4 h; boxplot (median and interquartile range)
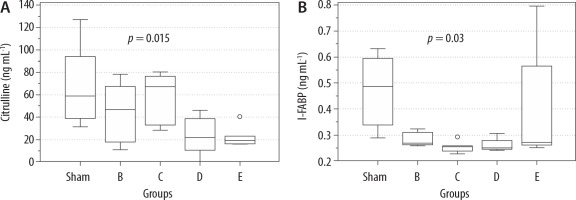
Regarding the ALT and AST measurements at the end of the experiment, no statistically significant differences were found among the groups. The median ALT in the sham group was 10.4 (7.4–22.9) IU L–1, in group B was 17.4 (7.4–18) IU L–1, in group C was 8.7 (7.7–9.9) IU L–1, in group D was 10.8 (7.0–21.3) IU L–1, and in group E was 10.1 (9.7–16.2) IU L–1; P = 0.88. On the other hand, the median AST in the sham group was 19.4 (10.0–27.2) IU L–1, in group B was 16.7 (12.8–31.5) IU L–1, in group C was 21.0 (14.0–23.5) IU L–1, in group D was 14.9 (12.3–19.5) IU L–1, and in group E was 14.4 (10.5–20.5) IU L–1; P = 0.66.
There were no differences among the groups in histological findings in any tissue or in the wet-to-dry ratios (Table 3).
TABLE 3
Total water gain (%) in organs of all groups
Discussion
Our study showed that both norepinephrine and vasopressin, combined or not with inotropes, were effective in the maintenance of MAP during endoto-xic shock, as demonstrated in an experimental study [22]. However, a greater reduction in Qao, a surrogate marker of CO, was observed with vasopressin than with norepinephrine. The regional blood flow, represented by the QSMA, also declined more significantly in the groups with vasopressin, which might be the possible cause of enterocyte dysfunction, as shown by lower serum citrulline levels in the sham group. It seems that vasopressin maintains MAP levels but at the expense of a reduction in CO (aortic flow), likely due to its potent vaso-constrictor effect. It was also demonstrated that along with lower CO values, worsening global perfusion resulted from reduced SvcO2 and increased serum lactate levels.
Vasopressin raises MAP by vasoconstriction, which is mediated primarily via stimulation of V1 receptors. However, the increase in the left ventricular afterload is associated with a reduction in CO with potential impairment of global and regional flow [23, 24]. Indeed, Sun et al. [22], in a model of sepsis induced by caecal ligation and perforation in sheep, found that vasopressin was able to maintain the target MAP but with lower CO and QSMA than when using norepinephrine. Westphal et al. [25], in an experimental model of septic shock induced by the same model in rats, evaluated splanchnic flow by intestinal video microscopy after resuscitation with vasopressin or fluids, and the results showed that animals receiving vasopressin exhibited less continuous flow, lower erythrocyte velocity, longer periods of microvascular circulatory arrest and, consequently, lower mean microcapillary blood flow in comparison to fluids alone. Accordingly, in a prospective study in 11 patients with septic shock receiving vasopressin, the authors correlated the serum level of vasopressin with its effects on splanchnic circulation estimated by gastric tonometry (PaCO2 gap). They detected a linear correlation between the serum vasopressin level and PaCO2 gap, reflecting a dose-related effect of vasopressin on gastric mucosa blood flow [26].
An experimental study [13] of endotoxic shock in rats showed that despite restored blood pressure, there was damage to the intestinal microcirculation. When dobutamine is combined with noradrenaline, there is a significant recovery from microcirculatory perfusion.
Another clinical study [27] with 42 septic patients showed the ability of dobutamine to significantly increase splanchnic DO2 and VO2 when compared to those without dobutamine. It is also important to note that there was no difference between 5 and 10 μg kg–1 min–1. It was concluded that the improvement in splanchnic perfusion was not observed at doses higher than 5 μg kg–1 min–1 of dobutamine. In our study, there was no significant difference between the blood flow of the superior mesenteric artery between the noradrenaline and noradrenaline + dobutamine groups, both of which remained stable. However, in the vasopressin and vasopressin + dobutamine groups, we observed a significant reduction in blood flow from the superior mesenteric artery and the absence of a protective effect of dobutamine in splanchnic perfusion.
Over the course of time, the difference in flow accompanied by changes in lactate is remarkable. Therefore, we consider the variation in the baseline to be physiological variability, as also seen in the Qao.
Superior mesenteric artery blood flow was lower in group A than in the other groups. In addition, arterial hypotension was present at least at 1 and 2 h. This was a possibly sedation effect representing only hypotension and not shock, as the lactate levels remained unchanged.
The increase in serum lactate level from baseline was more pronounced in the vasopressin group than in the other groups. Similarly, the study by Sun et al. [22] showed that serum lactate levels were significantly higher in these groups, showing worse global perfusion in addition to worse regional perfusion. In this regard, we observed that there was also an increase in lactate in the other two groups (B and C). In addition to sepsis, there may possibly be an increase in lactate due to the sources of aerobic production. This is a condition known to be present in septic shock, for example, due to the increase in aerobic glycolysis secondary to endogenous or exogenous catecholamines [28].
We did not observe signs of gut ischaemia, as demonstrated by the serum levels of I-FABP, either with norepinephrine or with vasopressin [29, 30]. In addition, AST and ALT were not significantly altered in this model of endotoxic shock, which may be related to the short observation period of only 4 h after the injection of LPS.
The analysis of the intestinal biomarkers showed worsening of enterocyte function (lower levels of citrulline) with the use of vasopressin, and significantly lower levels in I-FABP in the groups that used vasopressors as compared to the sham group. In other studies [29, 30], I-FABP level was significantly higher in patients with intestinal ische-mia. Splanchnic hypoperfusion, associated with the direct effect of sepsis, can lead to enterocyte dysfunction [31]. There are several definitions of gastrointestinal tract dysfunction, which makes it difficult to diagnose it in critically ill patients. Experimental and clinical studies have shown that some biomarkers may help in the identification of alterations in the gastrointestinal tract, including citrulline, whose reduction indicates enterocyte dysfunction, and I-FABP, which is a marker of ischae-mic injury to enterocytes [31].
In our study, citrulline levels were significantly lower in animals receiving vasopressin than in the control group, which may be due to the greater reduction in splanchnic flow in these groups, given that those receiving norepinephrine also had endotoxic shock. The evidence from the literature supports our findings because, in the case of septic shock, enterocyte dysfunction by the direct effect of sepsis is marked by reduced serum citrulline levels [32]. In addition, low blood flow may be a secondary factor in enterocyte dysfunction [33].
The I-FABP levels in our study were significantly lower among the experimental groups compared to the control group, which should be interpreted as the absence of ischaemic necrosis as indicated by the negative predictive value of this test [29, 30]. Possible causes for the divergence from the lite-rature, where conditions of low intestinal blood flow showed an increase in I-FABP [29], may be the ade-quate fluid resuscitation of all animals studied and the short duration of the experiment. There is no lite-rature that defines I-FABP level in rabbits, and perhaps for this reason it had lower values in our study. In addition, as all animals were resuscitated, the change in flow may have led to posterior dysfunction, marked by citrulline, but without the presence of sufficient necrosis to increase the I-FABP level.
Strengths and limitations of the study
One strength of our study was the attempt to simulate an ideal scenario of endotoxic shock in which vasopressors were used in volume-resuscitated individuals to avoid the effect of hypovolaemia or oedema as a confounding factor in tissue hypoperfusion.
The main weakness was that citrulline and I-FABP were only collected at the end of 4 hours, and this may have hampered the interpretation of these biomarkers. However, we used the sham group as a reference and the animals all underwent the same surgical procedure and the only differences were endotoxic shock and vasoactive drugs, comparison between groups at the end of 4 hours could identify possible differences in this matter. In addition, the short observation time may not be sufficient to identify more lesions over time, but despite the short observation period, important changes were noted in intestinal injury and function biomarkers. Another important limitation was the lack of a factorial design to separately study the effects of dobutamine, a vasopressor control group without endotoxin or a control group without resuscitation after endotoxin administration. Furthermore, at baseline, the sham group presented blood flow in the superior mesenteric artery that was quite different compared to the other groups, although it remained stable. Furthermore, other factors can influence the levels of these biomarkers in humans, such as kidney damage, and the serum levels of biomarkers in animals are not well known [10]. Finally, the dosages of vasopressors/inotropes were not reported at each time point for each group; only the doses with the average of the values used were described, because the aim of the study was to test the perfusion effect of medications.
CONCLUSIONS
Vasopressin was more effective in maintaining MAP during endotoxic shock in rabbits, but it reduced global and splanchnic blood flow, despite the association of an inodilator and adequate fluid replacement. These results may be a discriminator for the choice of appropriate treatment with vasopressors for patients with distributive shock.