Purpose
Brachytherapy (BT) plays an important role in the radiation treatment of vaginal tumors. Primary and recurrent cancers in the vagina are often treated with a BT boost after external beam radiotherapy (EBRT) to the pelvis [1-3]. Due to the diversity of location of vaginal tumors, vagina brachytherapy often faces challenges.
In brachytherapy, achieving high-dose conformability requires that the applicator and interstitial needles have to conform to certain arrangement rules, such as the Paris system. 3D-printed vaginal cylindrical template (3D-PVCT) due to its biodegradability, biocompatibility, and magnetic resonance (MR) compatibility, is suitable for guiding the accurate implantation of interstitial needles into tumors, helping in highly conformal dose distribution [4-8]. However, all 3D-PVCTs are personally designed, which poses a challenge for young physicians or residents with less experience. The purpose of this study was to present a detailed description of a novel technique involving the use of a 3D-PVCT with curved needle channels for delivering brachytherapy treatment to patients with vaginal tumors. This technique represents a significant advancement in the field of brachytherapy, as it allows for precise and targeted delivery of radiation to the affected area in completely different patients.
Material and methods
3D-PVCT
SketchUp 2022 (Google Inc., California, the United States) is an extremely popular and easy-to-use 3D design software. 3D-PVCT was designed using SketchUp 2022 based on our previous research [5]. A 3D-PVCT is composed of three parts. A cylindrical body with a curved top with a diameter of 3.0 cm and length of 14.0 cm is divided into main body (part one) and plug-in (part two). The plug-in can be removed to make room for the implantation of tandem through cylindrical body. The diameter of 3.0 cm has been validated in our previous research, and is suitable for most Chinese patients. To control the depth of vaginal cylinder implantation and fix onto the vaginal cylinder, a perineal stopper (part three) is connected to the cylinder that may adjust installation position.
Inside a 3D-PVCT, there are 23 needle channels, of which 7 are straight channels, with 2 of them located in the plug-in, and 16 are curved needle channels. Needle channels are modeled to have an inscribed hexagon shape with a diameter of 3 mm. The maximum deflection angle of all needle channels is 15 degrees, and the turning radius is greater than 38 mm. It is important to note that a 3D-PVCT is used only as a supportive guide template for the needles. Curved needles were plastic ProGuide needles (Interstitial Needle 083.066; Nucletron B.V., Veenendaal, The Netherlands), while straight needles were titanium interstitial needles (Titanium Needle Set 110.020; Nucletron B.V., Veenendaal, The Netherlands).
For those interested in obtaining 3D-PVCT, a 3D printer executable file (*.STL) can be downloaded from Supplemental File 1. However, before applying a 3D-PVCT to patients, it is crucial to verify the smoothness of all needle channels using actual needles and iridium-192 (192Ir) afterloading source. To ensure the safety of patients, the 3D-PVCT is made of a biocompatible material, and molecular structure is polylactic acid. This material has been deemed safe for placement inside the patient’s body. The 3D-PVCT needs to undergo low-temperature disinfection with 55 degrees Celsius ethylene oxide to be aseptically implanted within the patient. In order to illustrate the direction of each needle channel in the 3D-PVCT, a demo application was provided in Figure 1. The demonstration shows ten interstitial needles passing through the 3D-PVCT.
Patient No. 1
A 65-year-old woman had undergone hysterectomy due to adenomyosis, which was characterized by the presence of endometrial tissue within the muscular walls of the uterus. However, six years later, she was admitted to the hospital due to experiencing irregular vaginal bleeding. After MR examination, a tumor measuring 5.2 cm × 3.0 cm × 5.2 cm was discovered in the vaginal cuff in the upper third of the vagina (as shown in Figure 2). Pathological examination revealed squamous cell carcinoma. The patient was scheduled to receive whole pelvis EBRT, with the dose of 45 Gy in 25 fractions, weekly concomitant cisplatin (40 mg/m2), combined with MR image-guided BT (MR-IGBT) of 28 Gy in four fractions, two fractions within one application with at least 12 hours interval between two fractions, and at least one week interval between two applications.
Fig. 2
Pre-treatment magnetic resonance images of patient No. 2. A) T2 fast spin echo (FSE) transverse section, B) T2 FSE sagittal section, C) T2 FSE coronal section, and D) T1 FSE coronal section. White arrow represents the margin of vaginal stump tumors
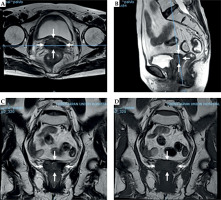
After EBRT delivered, a pre-plan process of 3D-PVCT-based BT was carried out. The pre-plan process is a step-by-step procedure to ensure accurate dose distribution prediction, and it is described as follows:
Vaginal cylinder with the same diameter as the 3D-PVCT is inserted into the vagina to shape the topography of actual treatment. Creating a realistic representation of the treatment area helps to accurately predict dose distribution.
MR scan is performed to identify and outline target volumes and organs at risk (OARs). High-risk clinical target volume (HR-CTV) is defined as the macroscopic gross tumor that remains at the time of brachytherapy as indicated by clinical examination and imaging, including any abnormal signal within the initial tumor extent. Intermediate-risk clinical target volume (IR-CTV) is determined by adding a minimum safety margin of 5 mm to HR-CTV, and including the initial extent of disease at diagnosis and one-third of the vagina. It helps to account for any potential microscopic tumor spread and ensure adequate coverage. OARs include the bladder, rectum, sigmoid, and small bowel.
Computed tomography (CT) image set of the 3D-PVCT with dummy interstitial needles is registration to MR image.
Based on the relationship between the tumor and the demonstrated 3D-PVCT, an appropriate angle for 3D-PVCT implantation and suitable needle channels are selected to ensure an optimized position distribution of each needle in the tumor, as presented in Figure 3.
Based on the chosen angle and needles, a pre-plan for the treatment is created and optimized. Linear quadratic model (LQ) is used to calculate the equivalent dose in 2 Gy per fraction (EQD2), with α/β = 10 for the tumor and α/β = 3 for OARs. Referring to the prescription doses specified in the EMBRACE II study, the total EQD2 dose summed from EBRT and BT to HR-CTV is set at D90 ≥ 85 Gy, while the total dose to IR-CTV is set at D98 ≥ 65 Gy [9]. Dose constraint for OARs is also based on the guidelines recommended in the EMBRACE II study [9].
If necessary, adjustment of the implantation angle of the 3D-PVCT and/or re-selection of the needles are made for comparing the optimal positioning of the needles for minimal insertion and efficient dose delivery.
The chosen angle of 3D-PVCT, needles, and implantation depth of each needle are recorded as a reference for ultrasound to assist in guiding the actual process of implantation.
Fig. 3
Simplified diagram of the relationship between tumor and demo 3D-printed vaginal cylindrical template. A) Aerial view and B) bottom view. Circles in green (four) are the selected curved needle channels, and squares in blue (five) are the selected straight channels. Light yellow represents vaginal stump tumors
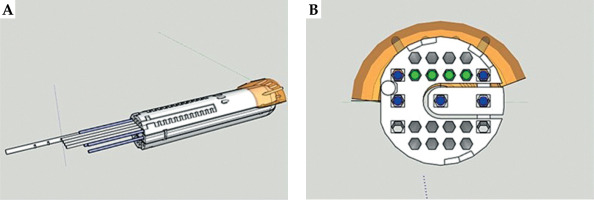
During the actual process of implanting 3D-PVCT and needles, the patients were under general anesthesia and real-time guidance of trans-rectal ultrasound was applied according to the pre-determined treatment plan. A total of nine needles were inserted into the tumors located in the anterior upper vaginal wall. Out of these nine needles, four were curved needle channels, and the remaining five were straight channels. The total insertion time for patient No. 1 was 19 minutes.
Patient No. 2
A 52-year-old woman with stage IB1 cervical cancer, who initially underwent laparoscopic hysterectomy, bilateral salpingo-oophorectomy, and pelvic lymphadenectomy. Upon pathological examination, she was diagnosed with squamous cell carcinoma, with no lymph node metastases and negative vaginal margins. As a result, adjuvant chemo/radiotherapy was not required. However, three years later, a recurrence was detected in the right side of the vaginal cuff (Figure 4). In order to address the recurrence, the patient was scheduled to receive whole pelvis EBRT with concurrent chemotherapy combined with MR-IGBT.
Fig. 4
Pre-treatment magnetic resonance images of patient No. 1. A) T2 fast spin echo (FSE) transverse section, B) T2 FSE sagittal section, C) T2 FSE coronal section, and D) T1 FSE coronal section. White arrow represents the margin of vaginal stump tumors
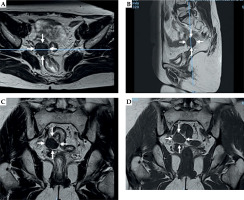
After completing EBRT, a pre-plan process of 3D-PVCT-based BT was described the same as for patient No. 1. During the pre-plan process, the angle of insertion for 3D-PVCT was determined. The appropriate needle channels were selected based on the relationship between tumor and demo 3D-PVCT, as shown in Figure 5.
Fig. 5
Simplified diagram of the relationship between tumor and demo 3D-printed vaginal cylindrical template. A) Aerial view and B) bottom view. Circles in green (six) are the selected curved needle channels, and square in blue (one) is the selected straight channel. Light yellow represents vaginal stump tumors
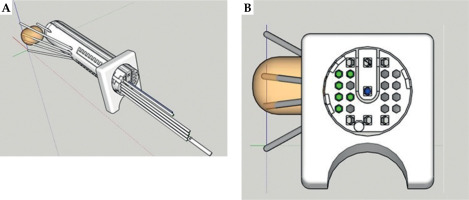
During the actual process of implanting 3D-PVCT and needles, the patient was under general anesthesia, and seven needles were inserted into the tumors located at the vaginal cuff with the guidance of trans-rectal ultrasound. Out of these seven needles, six were curved needle channels, and one was a straight channel. The total insertion time for patient No. 2 was 14 minutes.
Results
Two patients underwent a treatment regimen consisting of EBRT with concurrent chemotherapy combined with MR-IGBT. The EBRT was delivered in 25 fractions over a total dose of 45 Gy. The MR-IGBT was administered in four fractions with a total dose of 28 Gy, utilizing two fractions per application with a 12-hour interval between them. During the actual treatment, the patients were under general anesthesia and real-time guidance of trans-rectal ultrasound was followed according to the pre-determined treatment plan. Gradual insertion of the needles into the tumors was carried out during the procedure. Afterwards, MR imaging was done with the applicator in situ to perform the actual treatment planning. Both patients exhibited conformal dose distribution, as depicted in Figure 6. Doses delivered to the target volume and organs at risk (OARs) of both patients met dose constraints specified in the EMBRACE II guidelines, as outlined in Table 1. Currently, at the time of writing the manuscript, patient No. 1 has been followed up for 15 months, while patient No. 2 has been followed up for 18 months. Both patients have shown complete response to the treatment, with no evidence of tumor recurrence. Additionally, there have been no significant reports of acute or long-term toxicities.
Fig. 6
Dose distribution for the two patients. A) Transverse, B) sagittal, and C) coronal sections of patient No. 1; D) transverse, E) sagittal, and F) coronal sections of patient No. 2
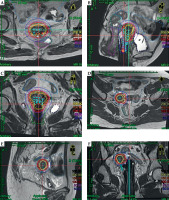
Table 1
Dose to target volumes and organs at risk
[i] BT – brachytherapy, EQD2 – equivalent dose in 2 Gy per fraction, HR-CTV – high-risk clinical target volume, D90 – minimum dose delivered to 90% of target volume, D98 – minimum dose delivered to 98% of target volume, IR-CTV – intermediate-risk clinical target volume, D2cc – minimum dose to 2 cm3 volume of OAR that received maximum dose
Discussion
Brachytherapy is a crucial component of radiotherapy, and it actually predates EBRT, having been used for more than 120 years. Brachytherapy has particularly significant implications in radical treatment of cervical cancer. Numerous studies and datasets have demonstrated that the addition of BT to cervical cancer treatment greatly enhances both the overall survival rate and cancer-specific survival [10, 11]. The effectiveness of BT heavily relies on the distribution of absorbed dose in the tumor, which is directly influenced by topography between the applicator or interstitial needles and the tumor. In the era of low-dose-rate line source treatment, the distribution of interstitial needles should adhere to the Paris system guidelines. This system specifies that the line sources should be arranged in a parallel, equidistant pattern, either in equilateral triangle or square configuration [12]. However, with the introduction of high-dose-rate treatment using a single-point source, the dwell time intensity can be conformally modulated and optimized accordingly. As a result, the distribution of interstitial needles can deviate slightly from the strict Paris system guidelines. This means that oblique needles with a specific angle or curved needles are permitted [4, 5, 7, 8].
Guide templates are widely used in brachytherapy to maintain a reasonable arrangement between interstitial needles. These supportive guide templates can be based on ovoids, rings, or cylinders, which play a crucial role in ensuring that the insertion points and insertion angles of each interstitial needle are in control [13-15]. Control level is essential for precise and accurate treatment.
The use of 3D printing additive manufacturing in radiation oncology showed significant advancements. Various applications, such as quality assurance phantoms, brachytherapy applicators, patient-specific bolus, and patient immobilization have benefited from the 3D printing technology [16-18]. In particular, 3D-PVCTs have been widely applied to assist in the rational distribution of interstitial needles curing vaginal tumors. However, it should be noted that most of these 3D-PVCTs are custom-designed for individual patients, or are based on complex inverse design principles [4, 5, 7, 8]. This customization allows for a channel selective tailored approach to each patient’s unique anatomy, increasing accuracy and effectiveness of treatment.
In a study conducted by Sekii et al., personalized template-guided interstitial brachytherapy was performed in two patients with vaginal tumors using 3D printing technology [19]. The researchers used a technique called ‘inverse design’ in their 3D-PVCT, which resulted in a remarkable dose distribution without causing any grade 3 or higher toxicity in patients.
Another study conducted by Laan et al. focused on developing personalized brachytherapy applicators using 3D printing technology [8]. These applicators were customized according to the individual vaginal topography of patients and included guided needle channels. The applicators also featured curved needle channels that could be used for both intracavitary and guided interstitial needle placement.
In our previous study, we presented an individualized 3D-PVCT, a 3D-printed multichannel with curved guide holes for interstitial needles specifically designed for vaginal tumors [5]. We conducted a pictorial essay showing successful implementation of this technique and positive clinical outcomes. The personalized 3D-PVCT utilized in our study demonstrated excellent dosimetric distribution.
However, it is important to note that the use of personalized 3D-PVCTs requires healthcare practitioners to possess a certain level of expertise in 3D modeling, inverse design, and 3D printing technology. This technology is still relatively new and may necessitate specialized training for its effective utilization.
In the current study, the main goal was to develop a 3D-PVCT that could be universally applicable for the treatment of vaginal cuff tumors. We selected two patients with vaginal cuff tumors, who had varying positions and shapes, highlighting the need for a customizable approach to treatment. To address this challenge, we applied a pre-plan technique to optimize template implantation angle and to select appropriate interstitial needle. This involved careful analysis of the patient’s anatomy and tumor’s characteristics to ensure optimal positioning and targeting. During the implementation of the approach, we noticed some variations between pre-planned template angle and actual treatment angle as well as differences in tumor target delineation. However, despite these disparities, we were pleased to observe that the overall results met the necessary dosimetric requirements. This indicates that our design approach has considerable robustness, as it can still achieve effective tumor targeting and deliver the required radiation dose, even in essential variations.
The 3D-PVCT is a unified designed template that fit the anatomy of each patient’s vaginal cuff tumors. It is created using 3D printing technology, which ensures a high level of accuracy and standardization. One of the key features of 3D-PVCT is the inclusion of curved needle channels. These channels are strategically positioned within the template to accommodate the vaginal cuff. This enables the brachytherapy needles to be inserted at the optimal angles and positions for effective treatment delivery. The curved needle channels also help to minimize trauma to the surrounding healthy tissues and organs. The usage of 3D-PVCT offers numerous advantages over traditional implantation [20]. Firstly, it improves the efficiency and accuracy of the treatment, as the template ensures precise needle placement. This reduces the risk of incomplete or excessive radiation doses to the tumor, maximizing the therapeutic effect. Additionally, the template protects healthy tissues from radiation damage, minimizing side effects and complications. Furthermore, the use of 3D printing technology allows for rapid production of a 3D-PVCT, making it readily available for widespread clinical application.
Currently, there are two interstitial needle insertion approaches for the treatment of vaginal cuff tumors, namely trans-vaginal approach and trans-perineal approach. Needle insertion for the trans-vaginal approach is easy because the needle path is short, and deep sedation is not always required. For the trans-perineal approach, wider range of needle insertion is possible due to its flexible angulation; however, some implantation paths may not be available due to block of bone [21]. Sekii et al. reported that inserting needles through the vagina reduces pain compared with inserting needles through the perineum [19]. Therefore, in institutions with a shortage of anesthesiologists, the implantation of 3D-PVCT through the vagina is beneficial for pain reduction in patients under local anesthesia.
Target definition of brachytherapy for vaginal cancer had always been a focus, and in October 2022, brachytherapy target definition guidelines for recurrent endometrial and cervical tumors in the vagina were published [22]. The guidelines provided definitions and comments on gross tumor volume (GTV)res, clinical target volume (CTV)-THR, and CTV-TIR.
The clinical practice of the two patients reported in this article adopted the naming and definition of GTV and CTV, as their treatments predated the guidelines. Based on our clinical definition and the GEC-ESTRO (ACROP) – ABS-CBG consensus, we believe that the target volume referred to as GTV in our clinical practice corresponds to HR-CTV in this guideline, while CTV corresponds to IR-CTV. Slightly different, we included one-third of the vagina in IR-CTV to consider the potential spread of tumors.
The application of the dose constraints from the EMBRACE II protocol to the two patients with vaginal cancer in this study has certain applicability. The dose constraints for OARs from the EMBRACE II protocol, such as rectum D2cc, sigmoid D2cc, and bladder D2cc, were based on previous evidence-based research results, showing universal applicability under the same radiation type. Additionally, these dose constraints are in line with the American Brachytherapy Society consensus guidelines for interstitial brachytherapy in vaginal cancer [23]. Therefore, it is feasible to apply the dose limit values of the EMBRACE II study to vaginal cancer.
In terms of target volume dose constraints, in 2012, the American Brachytherapy Society consensus guidelines for interstitial brachytherapy for vaginal cancer suggested that the total EQD2 should be in the range of 70 to 85 Gy [23]. However, Dimopoulos et al. adopted a higher D90, with a maximum D90 of 110 Gy, a median and interquartile range of 88 Gy and 78 Gy to 93 Gy, respectively, achieving a high 3-year overall survival rate of 85% [24]. In contrast, Lee et al. used a moderate D90 with an average of 74 Gy, achieving only a two-year overall survival rate of 62% [25]. A review published by Westerveld et al. in “Lancet Oncology” in 2020 reported that primary vaginal cancer is a rare cancer, and clinical evidence to support recommendations on its optimal management is insufficient. Since primary vaginal cancer resembles cervical cancer in many aspects, treatment strategies are mainly adopted from evidence in locally advanced cervical cancer [26]. Therefore, in our clinical work, the EMBRACE II dose constraints were commonly used for the dose in a primary vaginal cancer. Only when the dose to OARs exceeds the dose constraints, the dose to target volume can be considered reduced to protect OARs.
In the radical radiotherapy of cervical cancer, there are significant dose effect relationships between tumor control rate and doses to tumors [13, 27-30]. This study referenced and achieved the limits and planning aims from the EMBRACE II study [9]. Based on the cognition of dose effect relationship and combined with the dose results of our two patients, it can be preliminarily evaluated that this 3D-PVCT has certain clinical application value.
Our study has the following major limitations. First, the 3D-PVCT was used in only two patients with initial application. Although the tumor sites of the two patients were completely different, it does not mean that they can be universally applicable to all patients with vaginal cuff tumors. The current study presents only the first step towards establishing a universal 3D-PVCT, whereas extensive subsequent studies are needed to optimize the needle channels for universal application in clinical practice with more patients. Second, the curved needles based on the 3D-PVCT were inserted into tumors from the upper third of the vagina, so it is not suitable for patients with tumors in the middle and even lower third of the vagina. Third, in our study, the 3D-PVCT was only one size, and we hope to construct several different sizes of 3D-PVCT in the next stage of research to ensure the flexibility of use in patients with different vaginal laxity conditions.
Conclusions
The 3D-PVCT with curved needle channels has advantages of degradability, non-toxic, and magnetic resonance compatibility during brachytherapy of vaginal cuff tumors. With sufficient pre-plan, good target coverage, and OARs sparing could be achieved in the two investigated patients, demonstrating the potential for flexibility and universality. The standardized nature of the template and the rapid production capabilities of 3D printing technology make it a promising option for future clinical applications.