Introduction
Acute myeloid leukemia (AML) is an aggressive hematological malignancy characterized by uncontrolled proliferation of immature myeloid cells [1]. From the genetic background and clinical manifestations, AML is a highly heterogeneous disease [2]. In recent years, with the development of chemotherapy, hematopoietic stem cell transplantation, immunotherapy and molecular targeted therapy, the prognosis of most patients with AML has substantially improved [3]. However, 50% to 60% of patients still relapse, without long-term disease-free survival [4]. Studies have shown that drug resistance is the key to treatment failure, which leads to short-term survival of AML [3, 5]. The mechanism of drug resistance in AML is still unclear, which may be the result of a combination of factors [3]. Therefore, it is necessary to explore novel biomarkers for the diagnosis and treatment of drug resistance in AML in order to develop more effective monitoring and treatment programs.
Non-coding RNA (ncRNA) is an emerging tool for diagnosis, prognosis, and treatment of multiple hematological malignancies, including AML [6, 7]. Circular RNA (circRNA) is one class of widely distributed and highly stable ncRNA, well known for its circular closure structure [6]. CircRNA is derived from linear mRNA by back-splicing, and its stability is due to the lack of a 3' and 5' terminal, so it is more likely to be used as a marker in different human diseases [8]. The involvement of circRNAs is documented in numerous human malignant tumors, including liver cancer, lung cancer and bladder cancer [9]. A growing number of circRNAs are documented to be aberrantly regulated in AML and emerged to be promising biomarkers in AML diagnosis and therapy, such as circANAPC7, circ-VIM and circDLEU2 [10-12]. However, research on circRNAs in AML chemoresistance is still lacking. Circ-NPM1 (circ_0075001), generated from NPM1, was reported to play a crucial role in AML [13]. We focused on this circRNA and explored its detailed functions in AML cells.
MicroRNA is also a class of ncRNA, approximately 22 nucleotides in length [6]. Widespread dysregulation of miRNAs has been found in AML, and alteration of mi-RNA expression may trigger AML deterioration or alleviation [6, 14]. Previous reports illustrated that miR-345 was dysregulated in bone marrow from AML patients [15], suggesting that miR-345 might be involved in AML progression. However, related studies that explain the function and mechanism of miR-345 are limited.
Frizzled-5 (FZD5) is a member of the frizzled protein family and serves as a receptor of Wnt proteins [16]. The Wnt signaling pathway is an important axis in tumorigenesis and development, and FZD5 associated with the Wnt pathway participates in the progression of diverse cancers [17, 18]. Whether FZD5 also plays a key role in AML is worth exploring.
In this study, we investigated the function of circNPM1 in Adriamycin (ADM)-treated AML cells to observe the role of circNPM1 in chemoresistance. Additionally, the related regulatory mechanism of circNPM1 associated with miR-345-5p and FZD5 was expounded. Our study aimed to determine the function of circNPM1 in AML and provide new insights into the development of drug resistance in AML.
Material and methods
Serum samples
AML patients (n = 20) and healthy volunteers (n = 20) were recruited from Jingjiang Chinese Medicine Hospital. The peripheral blood samples were collected from each subject, and serums were obtained from peripheral blood by centrifugation. Informed consent was obtained from all subjects before sample collection. Serum samples were preserved at –80°C storage. This study was approved by the Ethical Committee of Jingjiang Chinese Medicine Hospital.
Cell lines and ADM treatment
Experimental cells, including human AML mononuclear cells (THP-1) and human promyelocytic leukemia cells (HL-60), and control cells, including marrow stromal cells (HS-5), were all purchased from Bena Culture Collection (BNCC; Beijing, China). THP-1 and HL-60 cells were cultured in 90% Roswell Park Memorial Institute 1640 (RPMI 1640; BNCC) + 10% fetal bovine serum (FBS), and HS-5 cells were cultured in 90% Dulbecco modified Eagle medium (DMEM; BNCC) + 10% FBS. All cells were cultured in a 37°C incubator mixed with 5% CO2. Partial cells were exposed to 5 µg/ml ADM for 48 h before cell transfection.
Quantitative real-time polymerase chain reaction
Total RNA was extracted from serum samples and cells using TRIzol reagent (Invitrogen, Carlsbad, CA, USA). RNA samples were tested and assembled into cDNAs using the First Strand cDNA Synthesis Kit (Thermo Fisher Scientific, Waltham, MA, USA). Next, qRT-PCR was conducted using SYBR GreenER qPCR SuperMix (Invitrogen) with a PCR system (Bio-Rad, Hercules, CA, USA). Glyceraldehyde 3-phosphate dehydrogenase (GAPDH) and U6 were utilized as internal references. The delta-delta cycle threshold (ΔΔCt) method was used for quantification. The primers were as follows: circ-NPM1, F: 5'-GCGCCAGTGAAGAAAGTTGT-3' and R: 5'-AAGGCTGAAATCAAGTAGGGTT-3'; miR-345-5p: 5'-GCTGACTCCTAGTCCA-3' and R: 5'-TGGTGTCGTGGAGTCG-3'; FZD5: 5'- TACCCAGCCTGTCGCTAAAC-3' and R: 5'- AAAACCGTCCAAAGATAAACTGC-3'; U6, F: 5'-CTCGCTTCGGCAGCACA-3' and R: 5'-AACGCTTCACGAATTTGCGT-3'; GAPDH, F: 5'-AGGGCTGCTTTTAACTGGT-3' and R: 5'-CCCCACTTGATTTTGGAGGGA-3'.
Subcellular distribution
Cellular fractionation was carried out using the PARIS Kit (Ambion, Austin, TX, USA) to isolate cytoplasmic and nuclear RNA. Cytoplasmic RNA and nuclear RNA were subjected to qRT-PCR to detect the expression of circNPM1 with GAPDH and U6 as the internal references, respectively.
RNase R treatment
RNA samples were treated with 3 U/µg RNase R (Epicentre, Madison, WI, USA) for 15 min at 37°C before reverse transcription. After that, RNA was purified using the RNeasy MinElute cleanup Kit (Qiagen, Duesseldorf, Germany) followed by detection using qRT-PCR to determine the expression of circNPM1 and linear mRNA NPM1.
RNA oligonucleotides, plasmids and cell transfection
Small interference RNA targeting circNPM1 (si-circ-NPM1) (RiboBio, Guangzhou, China) was used for circ-NPM1 silence, and si-NC (RiboBio) served as the control. MiR-345-5p mimics (miR-345-5p) (RiboBio) or miR-345-5p inhibitors (anti-miR-345-5p) (RiboBio) were used for miR-345-5p restoration or inhibition, and miR-NC or anti-miR-NC served as the respective control. A fusion plasmid of the pcDNA3.1 vector containing the FZD5 fragment (FZD5) (Sangon, Shanghai, China) was used for FZD5 overexpression, and an empty pcDNA vector (vector) (Sangon) served as the control. All of them were introduced into HL-60 and THP-1 cells using Lipofectamine 3000 (Invitrogen).
Colony formation assay
Transfected THP-1 and HL-60 cells were seeded into 6-well plates (1000 cells/well) and cultured for approximately 12 days at 37°C to allow colony growth. The colonies were fixed with 4% paraformaldehyde, stained with 0.1% crystal violet and photographed. The number of colonies was counted under a microscope (Olympus, Tokyo, Japan).
Flow cytometry assay
Cells were harvested by centrifuging and washed twice with phosphate-buffered saline (PBS). For apoptosis analysis, 1 × 106 cells were treated with 5 µl of Annexin V-FITC and 10 µl of propidium iodide from a commercial kit (Beyotime, Shanghai, China), and cultured for 15 min in the dark. For cell cycle analysis, cells were fixed with 70% ethanol at 4°C for 6 h. Afterwards, cells were exposed to propidium iodide staining buffer containing RNase A (10 mg/ml) for 30 min at 37°C in the dark. Finally, cell apoptosis or cell cycle distribution at the different stages was analyzed by a flow cytometer (BD Biosciences, San Jose, CA, USA).
Transwell assay
Cell migration and invasion were monitored using the 8 µm transwell chamber (Corning Incorporated, Corning, NY, USA). Partial chambers were treated with Matrigel (Corning Incorporated). 2 × 104 cells resuspended in fresh corresponding culture medium were placed into the upper chambers with or without Matrigel for invasion assay or migration assay, respectively. The lower chamber was supplemented with fresh culture medium to allow cell migration and invasion for 24 h. Eventually, the migrated or invaded cells were fixed using paraformaldehyde and stained with 0.1% crystal violet followed by photography under a microscope (Olympus).
Western blot
RIPA lysis buffer (Beyotime) was used for protein extraction. Then protein was treated according to the previous description [12]. The primary antibodies were anti-matrix metalloproteinase 2 (MMP2; ab97779; Abcam, Cambridge, MA, USA), anti-matrix metalloproteinase 9 (MMP9; ab76003; Abcam) and anti-FZD5 (ab75234; Abcam). Anti-GAPDH (ab9485; Abcam) was used as a loading control.
Bioinformatics analysis
The potential target miRNAs of circNPM1 or target mRNAs of miR-345-5p were predicted by the bioinformatics tool starBase (http://starbase.sysu.edu.cn/).
Dual-luciferase reporter assay
For detecting the interaction between circNPM1 and miR-345-5p, the wild-type sequences of circNPM1 harboring the binding sites with miR-345-5p and its corresponding mutated sequences were inserted into a pmirGLO vector (Promega, Madison, WI, USA), naming them as circNPM1 WT and circNPM1 MUT, respectively. Similarly, the 3' UTR of FZD5 harboring the miR-345-5p binding sites and its corresponding mutated sequence were also cloned into the pmirGLO vector, naming them as FZD5 3' UTR WT and FZD5 3' UTR MUT, respectively. Cells were transfected with these fusion plasmids and miR-345-5p or miR-NC, respectively. Luciferase activity was determined after 48-h transfection using the Dual-Luciferase Reporter Assay System (Promega).
RNA immunoprecipitation (RIP)
The Magna RIP Assay Kit (Millipore, Billerica, MA, USA) was used for RIP assay to determine the interaction between circNPM1 and miR-345-5p. According to the protocol, human Argonaute 2 (Ago2) was used for the experimental group, and mouse immunoglobulin G (IgG) was used as a negative control. After the reaction, the coprecipitated RNAs were extracted and detected by qRT-PCR.
Statistical analysis
The results were expressed as the mean ± standard deviation, and the data were calculated from at least three independent experiments. Student’s t-test was used to evaluate the statistical significance of differences between two groups, and significant differences among ≥ 3 groups were evaluated using analysis of variance with Tukey’s method. All data were processed using GraphPad Prism 5 software (San Diego, CA, USA). A p-value less than 0.05 was regarded as statistically significant.
Results
Expression of circNPM1 was increased in AML
We collected 20 serum samples from AML patients and healthy volunteers and found that circNPM1 expression was significantly increased in the AML serum compared to the healthy serum (Fig. 1A). Also, the expression of circNPM1 was notably enriched in THP-1 and HL-60 cells compared with that in HS-5 cells (Fig. 1B). To further determine the characteristics of circNPM1, we detected its distribution and stability. The data showed that circNPM1 was mainly distributed in the cytoplasm rather than in the nucleus (Fig. 1C, D), and circNPM1 was almost unaffected by RNase R relative to its linear mRNA (NPM1) (Fig. 1E, F). These findings suggested that circNPM1 was highly expressed in AML and was mainly distributed in the cytoplasm with a degree of stability.
Fig. 1
CircNPM1 was highly expressed in serum from AML patients and AML cells. A) Expression of circNPM1 in serum samples from AML patients and healthy volunteers was detected by qRT-PCR. B) Expression of circNPM1 in HS-5, THP-1 and HL-60 cells was detected by qRT-PCR. C, D) Nuclear and cytoplasmic RNAs were isolated to monitor the distribution of circNPM1 by qRT-PCR. E, F) RNase R was utilized to test the stability of circNPM1 and its linear mRNA. *p < 0.05
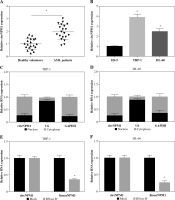
CircNPM1 silence impaired the resistance of AML cells to ADM and inhibited the malignant activities of AML cells
THP-1 and HL-60 cells were subjected to the transfection of si-circNPM1 and si-NC. To ascertain the transfection efficiency, we detected the expression of circNPM1 in transfected cells and found that circNPM1 expression was obviously lower in cells transfected with si-circNPM1 compared to si-NC (Fig. 2A, B), suggesting that the endogenous level of circNPM1 was effectively reduced in cells. For functional analysis, circNPM1 silence significantly weakened the ability of colony formation, and the ability of colony formation in cells treated with ADM and transfected with si-circNPM1 was noticeably lower compared with that in cells treated with ADM and transfected with si-NC (Fig. 2C, D). As expected, the apoptosis rate was strikingly higher in cells transfected with si-circNPM1 relative to si-NC, and also enhanced in cells treated with ADM and transfected with si-circNPM1 relative to si-NC (Fig. 2E, F). Also, circNPM1 silence led to cell cycle arrest at the G1-S transition stage, and si-circNPM1 transfection could enhance the effect of ADM to further block the cell cycle relative to si-NC transfection (Fig. 2G, H). In addition, the capacity of migration and invasion was markedly lower in cells transfected with si-circNPM1 compared with si-NC, and in the si-circNPM1-transfected cells treated with ADM, the migration and invasion potential was significantly lower compared to the si-NC-transfected cells treated with ADM (Fig. 2I-L). Additionally, we detected that the expression of MMP2 and MMP9 was weaker in cells transfected with si-circNPM1 relative to si-NC and also weaker in ADM-treated cells transfected with si-circNPM1 relative to si-NC (Fig. 2M, N). These data showed that circNPM1 silence could suppress the malignant activities of AML cells and enhance the sensitivity of AML cells to ADM.
Fig. 2
CircNPM1 silence impaired AML progression and enhanced the sensitivity of AML cells to ADM. A, B) THP-1and HL-60 cells were transfected with si-circNPM1 or si-NC, and the transfection efficiency was detected according tocircNPM1 expression by qRT-PCR. C) Cell proliferation ability was observed by colony formation assayD) Cell proliferation ability was observed by colony formation assay. E) Cell apoptosis was monitoredby flow cytometry. F) Cell apoptosis was monitored by flow cytometry.G) Cell cycle distribution was assessed by flow cytometry assay. H) Cell cycle distribution was assessedby flow cytometry assay. I) Cell migration and invasion were evaluated by transwell assayJ–L) Cell migration and invasion were evaluated by transwell assayM, N) Expression of MMP2 and MMP9 was measured by western blot. *p < 0.05
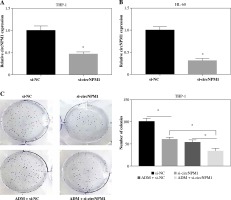
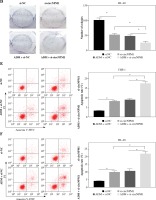
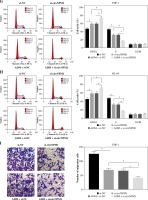
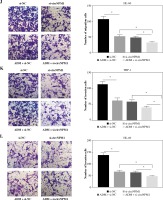
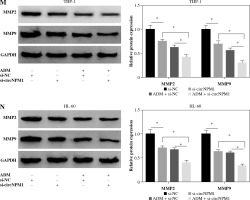
MiR-345-5p was a target of circNPM1 and was downregulated in AML
Next, we investigated the potential target miRNAs of circNPM1 so as to address its regulatory mechanism in AML. According to the bioinformatics analysis, miR-345-5p was a potential target of circNPM1 with special binding sites between their sequence fragments (Fig. 3A). To verify this prediction, dual-luciferase reporter assay and RIP assay were performed. The data showed that the luciferase activity was strikingly lower in cells cotransfected with miR-345-5p and circNPM1 WT relative to that in cells cotransfected with miR-NC and circNPM1 WT, while the luciferase activity showed no noticeable change in cells cotransfected with circ-NPM1 MUT and miR-345-5p or miR-NC (Fig. 3B, C). In addition, RIP assay demonstrated that both circ-NPM1 and miR-345-5p were abundantly detected in the Ago2 RIP group compared with the IgG RIP group (Fig. 3D, E). Moreover, the expression of miR-345-5p was substantially increased in THP-1 and HL-60 cells transfected with si-circNPM1 (Fig. 3F, G), suggesting that circNPM1 suppressed miR-345-5p expression. We also monitored the expression of miR-345-5p in serum samples and AML cells and found that miR-345-5p was significantly downregulated in the serum from AML patients and AML cells (THP-1 and HL-60) relative to healthy samples and HS-5 cells, respectively (Fig. 3H, I). These findings confirmed that miR-345-5p was a target of circNPM1.
Fig. 3
MiR-345-5p was a target of circNPM1. A) Bioinformatics tool starBase predicted the relationship betweencircNPM1 and miR-345-5p. B, C) Their relationship was verified by dual-luciferase reporter assay. D, E) Their relationshipwas also confirmed by RIP assay F, G) The effect of circNPM1 silence on the expression of miR-345-5p was checked by qRT-PCR.H) Expression of miR-345-5p in serum samples from AML patients and healthy volunteers was detected by qRT-PCR.I) Expression of miR-345-5p in HS-5, THP-1 and HL-60 cells was detected by qRT-PCR. *p < 0.05
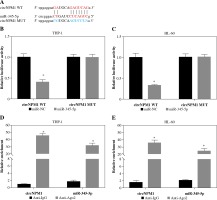
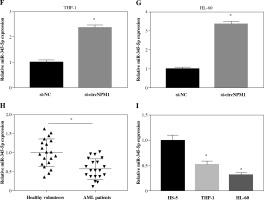
MiR-345-5p deficiency could reverse the effects of circNPM1 silence
Rescue experiments were performed to functionally explore the relationship between circNPM1 and miR-345-5p. Cells were introduced with different transfection, and the data from qRT-PCR showed that miR-345-5p expression was notably promoted in cells transfected with si-circNPM1 relative to si-NC but obviously reduced in cells transfected with si-circNPM1 + anti-miR-345-5p relative to si-circNPM1 + anti-miR-NC (Fig. 4A, B), suggesting that transfection was effective. Next, we transfected si-circNPM1, si-NC, si-circNPM1 + anti-miR-345-5p or si-circNPM1 + anti-miR-NC into ADM-treated THP-1 and HL-60 cells, termed the ADM + si-circNPM1 group, ADM + si-NC group, ADM + si-circNPM1 + anti-miR-345-5p group or ADM + si-circNPM1 + anti-miR-NC group. The number of colonies was visibly lower in the ADM + si-circNPM1 group compared to ADM + si-NC group but recovered in the ADM + si-circNPM1 + anti-miR-345-5p group relative to the ADM + si-circNPM1 + anti-miR-NC group (Fig. 4C, D). Also, cell apoptosis stimulated by circNPM1 silence was remarkably reduced by the introduction of anti-miR-345-5p in ADM-treated cells (Fig. 4E, F). Also, cell cycle arrest induced by circNPM1 silence was dramatically relieved by the introduction of anti-miR-345-5p in ADM-treated cells (Fig. 4G, H). Furthermore, cell migration and invasion notably inhibited in the ADM + si-circNPM1 group were strikingly stimulated in the ADM + si-circNPM1 + anti-miR-345-5p group (Fig. 4I-L). The expression of MMP2 and MMP9 was prominently lower in the ADM + si-circNPM1 group compared to the ADM + si-NC group but restored in the ADM + si-circNPM1 + anti-miR-345-5p group relative to the ADM + si-circNPM1 + anti-miR-NC group (Fig. 4M, N). These data indicated that miR-345-5p deficiency could partly reverse the functional effects of circNPM1 silence in AML cells.
Fig. 4
MiR-345-5p deficiency reversed the effects of circNPM1 silence in ADM-treated cells. THP-1 and HL-60 cellstreated with ADM were transfected with si-circNPM1, si-NC, si-circNPM1 + anti-miR-345-5p or si-circNPM1 + antimiR-NC. A, B) The transfection efficiency was checked according to miR-345-5p expression, and cellular activities,including C, D) colony formation, E, F) cell apoptosis. *p < 0.05G, H) cell cycle, I-L) migration and invasion, were investigated in these transfected cells to observethe function of circNPM1 and miR-345-5p. *p < 0.05M, N) Expression of MMP2 and MMP9 in these transfected cells was detected by western blot. *p < 0.05
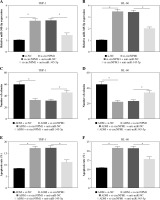
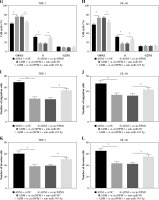
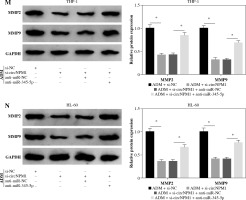
CircNPM1 targeted miR-345-5p to regulate the expression of FZD5
Subsequently, the putative target mRNAs of miR-345-5p were analyzed by the bioinformatics tool. As shown in Figure 5A, there were specific binding sites between FZD5 3'UTR and miR-345-5p sequence fragments (Fig. 5A). Then, the sequence of FZD5 3'UTR was mutated at the binding sites to perform the dual-luciferase reporter assay. The data revealed that miR-345-5p reintroduction notably diminished the luciferase activity in cells transfected with FZD5 3'UTR WT but not FZD5 3'UTR MUT compared to miR-NC (Fig. 5B, C). Also, miR-345-5p enrichment weakened the expression of FZD5 (Fig. 5D, E). Interestingly, we also noted that the expression of FZD5 was significantly lower in THP-1 and HL-60 cells transfected with si-circ-NPM1 relative to si-NC but higher in cells transfected with si-circNPM1 + anti-miR-345-5p relative to si-circNPM1 + anti-miR-NC (Fig. 5F, G). The expression level of FZD5 in AML serum samples and cells (THP-1 and HL-60) was also aberrantly enhanced compared with that in healthy serum samples and HS-5 cells, respectively (Fig. 5H, I). From the above data, we concluded that circNPM1 regulated the expression of FZD5 by targeting miR-345-5p.
Fig. 5
FZD5 was a target of miR-345-5p and was regulated by circNPM1 and miR-345-5p. A) The interaction betweenFZD5 and miR-345-5p was predicted by the bioinformatics tool starBase. B, C) The interaction between FZD5 and miR-345-5p was validated by dual-luciferase reporter assay. D, E) Expression of FZD5 in THP-1 and HL-60 cells with thetransfection of miR-345-5p or miR-NC was measured by western blot. *p < 0.05F, G) Expression of FZD5 in THP-1 and HL-60 cells with the transfection of si-circNPM1, si-NC, si-circ-NPM1 + anti-miR-345-5p or si-circNPM1+anti-miR-NC was evaluated by western blot. H, I) Expression of FZD5 inserum samples and cell lines was detected by western blot. *p < 0.05
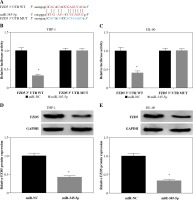
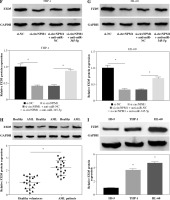
FZD5 could reverse the effects of miR-345-5p in AML cells
To further confirm that FZD5 was a downstream target of miR-345-5p, rescue experiments were performed. The data from western blot showed that FZD5 expression was blocked in cells transfected with miR-345-5p but visibly recovered in cells cotransfected with miR-345-5p + FZD5 (Fig. 6A, B), explaining that each transfection was available. In ADM-treated THP-1 and HL-60 cells, miR-345-5p transfection alone conspicuously impaired colony formation ability, while combined miR-345-5p and FZD5 transfection recovered colony formation ability (Fig. 6C, D). Certainly, cell apoptosis was notably stimulated by miR-345-5p transfection alone but repressed by miR-345-5p + FZD5 transfection in ADM-treated THP-1 and HL-60 cells (Fig. 6E, F). In addition, cell cycle arrest was triggered in ADM-treated cells transfected with miR-345-5p but blocked in ADM-treated cells transfected with miR-345-5p + FZD5 (Fig. 6G, H). Not surprisingly, cell migration and invasion were markedly restrained in ADM-treated cells with the transfection of miR-345-5p but promoted in ADM-treated cells with the transfection of miR-345-5p + FZD5 (Fig. 6I-L). Moreover, the expression of MMP2 and MMP9 was decreased by the transfection of miR-345-5p relative to miR-NC in ADM-treated cells but recovered by the transfection of miR-345-5p + FZD5 relative to miR-345-5p + vector (Fig. 6M, N). These functional analyses suggested that miR-345-5p mediated FZD5 to enhance the sensitivity of AML cells to ADM, thereby blocking the malignant properties of AML cells.
Fig. 6
FZD5 overexpression blocked the effects of miR-345-5p restoration in ADM-treated AML cells. THP-1 and HL-60cells were transfected with miR-345-5p, miR-NC, miR-345-5p + FZD5 or miR-345-5p + vector. A, B) Expression ofFZD5 in these transfected cells was detected by western blot to assess transfection efficiency. C, D) Cell proliferationwas examined by colony formation assay. *p < 0.05E, F) Cell apoptosis was analyzed by flow cytometry assay. G, H) Cell cycle distribution was determinedby flow cytometry assay. I, J) Cell migration and invasion were assessed by transwell assay. *p < 0.05K, L) Cell migration and invasion were assessed by transwell assay. M, N) Levels of MMP2 and MMP9were quantified by western blot. *p < 0.05
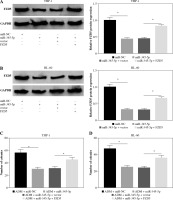
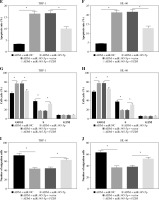
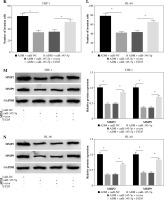
Discussion
Chemoresistance is one of the characteristics of AML that relapses after a brief remission following treatment, leading to resistance to further treatment [19]. Resistance to one drug can even lead to resistance to other drugs [20]. Therefore, exploring the mechanisms of development of drug resistance in AML and overcoming drug resistance will facilitate the treatment of AML.
Numerous circRNAs have been reported to be implicated in tumor initiation, chemoresistance to therapy, and hallmark, which was attributed to their abilities of gene regulation through diverse manners, including serving as microRNA sponges, and interacting with RNA-binding proteins [21, 22]. Recently, the mediation of circRNAs in drug resistance in AML attracts much attention. A previous study showed that circPAN3 was weakly expressed in chemo-sensitive AML patients compared to chemo-resistant AML patients, and circPAN3 knockdown suppressed the chemoresistance in AML cells to ADM by mediating the miR-153-5p/miR-183-5p-XIAP pathway [23]. In this study, we concentrated on circNPM1 (circ_0075001), which was revealed to be upregulated in leukemia cell lines compared to healthy controls [13]. The characteristics of circNPM1 were identified, and the data showed that circNPM1 was mainly located in the cytoplasm and resistant to RNase R. Functional experiments indicated that circNPM1 silence could strengthen the effects of ADM, leading to further cell proliferation, migration and invasion inhibition, apoptosis promotion and cell cycle arrest. It suggested that circNPM1 might be an effective regulator of ADM chemoresistance in AML cells.
To explore the potential mechanism of circNPM1 action and ascertain whether circNPM1 acted as a miRNA sponge to play its biological functions, we screened and identified its putative target miRNAs by bioinformatics analysis, dual-luciferase reporter assay and RIP. Of these miRNAs, miR-345-5p was previously illustrated to be downregulated in AML, and miR-345-5p restoration frustrated cell proliferation and induced cell apoptosis [24]. Also, the expression of miR-345 was proved to be reduced in acute lymphocytic leukemia samples [25]. These consequences suggested the important role of miR-345-5p in hematological malignancy. In agreement with these ideas, we detected that the expression of miR-345-5p was decreased in AML serum samples and cell lines. MiR-345-5p absence could reverse the effects caused by circNPM1 silence, and miR-345-5p restoration promoted ADM sensitivity in AML cells to alleviate malignant cellular activities.
Further, we found that FZD5 was targeted by miR-345-5p, and its expression was suppressed by miR-345-5p restoration. In addition, the expression level of FZD5 was aberrantly elevated in AML serum samples and cell lines. FZD5 was regarded as an oncogene in various cancers, such as hepatocellular carcinoma, prostate cancer and ovarian cancer [26-28]. Also, FZD5 was targeted by miR-212-5p to reverse the effects of miR-212-5p mimic in AML cells, resulting in the promotion of proliferation and the block of apoptosis [29]. In our study, we first investigated the role of FZD5 in chemoresistance in AML cells and discovered that FZD5 overexpression could block the role of miR-345-5p restoration to increase ADM resistance in AML cells, thus promoting AML malignant development. Moreover, we found that expression of FZD5 was reduced in cells by the transfection of si-circNPM1, while the transfection of miR-345-5p inhibitor enhanced FZD5 expression, indicating that FZD5 was a downstream molecule of circNPM1 and miR-345-5p.
Conclusions
Collectively, this paper for the first time discussed the role of circNPM1 in drug resistance in AML cells and concluded that circNPM1 contributed to ADM chemoresistance in AML cells partly by mediating the miR-345-5p/FZD5 regulatory axis. Our study highlighted that circ-NPM1 might be a potential biomarker in drug resistance therapy for AML.