Purpose
Synchronous chemoradiotherapy is the standard treatment for locally advanced cervical cancer (LACC), with 5-year survival rates ranging from 49% to 82% [1]. Due to the presence of natural ducts in the uterus, brachytherapy (BT) is the most suitable treatment for primary tumors that can achieve superior results using radiation source and high-intensity irradiation [2]. In recent years, along with the development of image-guided brachytherapy (IGBT) technology, rational selection and placement of applicators have become crucial to influence therapeutic effect of LACC.
Intracavitary brachytherapy (ICBT) is still one of the most commonly used methods for the treatment of LACC, with a local control rate ranging from 75% to 95% for small tumors [3]. When a tumor demonstrates a large volume, eccentric location, irregular shape, or invades parametrial or other sites, achieving an ideal dose distribution in ICBT becomes challenging. Intracavitary/interstitial brachytherapy (IC/ISBT) can effectively improve dose distribution for target of such tumors [4]. The operating method of IC/ISBT is to implant several needles into the tumor area to obtain better target dose coverage. Commercial IC/ISBT applicators or free-hand insertion are both choices for assisting this technology. However, commercial applicators, such as Utrecht and Vienna, have limited clinical applications due to their uniform size and unsuitability for patients. Free-hand method of operation relies heavily on a clinician’s experience, and often results in unfavorable needle positioning. Therefore, NCCN guidelines [5] emphasize that IC/ISBT therapy should only be administered in qualified medical facilities and by skilled personnel.
Individualized and customized three-dimensional (3D)-printed designs can address limitations of commercial applicators and guide IC/ISBT implantation. The utilization of 3D-printed templates has demonstrated the improvement in better dose distribution and operation time reduction [6], allowing for more favorable geometries [7] as well as facilitating individualization based on patient’s anatomy [8]. However, the clinical implementation of individualized 3D printing necessitates significant effort and time in developing customized templates [9]. The drawbacks primarily include: 1. Requirement for specialized technical expertise in 3D printing; 2. Access to high quality 3D printers, cost, and human resources for creating personalized devices per patient; and 3. Staff training on template prototyping. Therefore, the widespread application of customized 3D printing technology in IC/ISBT remains a challenge, particularly in developing countries. The 3D standardized template-guided IC/ISBT was proposed with no additional customization and printing process, and designed to allow more channel paths, increasing the flexibility of needle trajectories. Furthermore, the implementation of 3D standardized templates is mainly concerned with robustness of clinical use rather than biocompatibility, sterilization, manufacturing, and pre-planning in general customized 3D-printed templates.
To facilitate greater accessibility of IC/ISBT technique, the 3D standardized template was used to guide interstitial needles for optimal dosimetric positions across tumor topologies. We conducted this study to investigate the precision and consistency using a 3D standardized template.
Material and methods
Between September 2021 and December 2022, forty-three LACC patients at our department were enrolled in this study. All patients were diagnosed with FIGO 2018 stage IIB-IVA, and treated by external beam radiotherapy (EBRT) with 45-50.4 Gy and subsequent IC/ISBT with 28 Gy in 4 fractions (once a week). In terms of IC/ISBT, 24 patients were treated with 3D standardized templates (ST group), and 19 patients were treated using free-hand implantation (FH group). This study was approved by ethical committee of Women’s Hospital, School of Medicine Zhejiang University. Informed consent was obtained from all participants, and all methods were performed in accordance with relevant guidelines and regulations.
3D standardized template
The concept of this commercial 3D standardized template (FORRAD, China) aims to ease processing and allows precision of interstitial catheter placement during IC/ISBT to optimize tumor coverage, while decreasing dose to nearby normal tissues. Furthermore, templates are made to be compatible with standard applicator and designed based on common patient’s scenarios. Complete details of this template including development, beta-testing, and optimization of prototype design, have not been made public by the manufacturer. The cylinder of 3D standardized template includes several parts: 1. Central intracavitary applicator path; 2. 12 needle channels around the central path; and 3. Bilateral top and bottom, with 9 and 4 needle channels, respectively (Figure 1A). This 3D standardized template allows a wider scope of precise needle positioning and more degrees of freedom as needed for bulky tumors. The material of this template meets the standards of biocompatibility and sterilization, while the procedure using template-guided IC/ISBT is as follows: 1. Radiation oncologists determines the number and trajectory of needles on the template based on previous magnetic resonance (MR) images and gynecological examination; 2. After intracavitary applicator insertion into the uterus, the template with the arranged needles is implanted through an intrauterine tube into the vagina, 3. The template is immobilized through an in-house bandage attached from patient’s waist to the perinea (Figure 1B). The needles can be further adjusted without dismantling the apparatus post-procedure, and if desired, after obtaining initial scanning images.
Fig. 1
Examples of clinical implementation of 3D standardized template-guided IC/ISBT. A) Template with intracavitary applicator and inserted needles. B) Immobilization of the template after implantation using in-house bandage. C) Location of the template in the coronal section of CT scanning image. D) 3D vision after reconstruction of HR-CTV and applicator passage. Red refers to target volume, and green denotes template
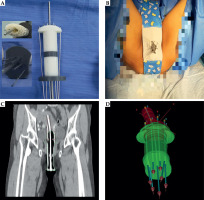
Clinical practice
For all patients, MR images were done 1 week before BT. Generally, the extent of implantation should be referred to both initial (pre-RT) and residual (post-RT) disease. Cleansing enema and oral contrast (diatrizoate meglumine) were required for preparations before each BT implantation session. In order to ensure the consistency of patient’s position between fractional BT, the transfer bed was used to record the location scale for each patient. In ST and FH groups, radiation oncologists decided the depth of intracavitary applicator and interstitial needles based on the volume, shape, and location of the tumor, and operated with CT guidance. When the entire implantation process was completed, the bladder was filled with 150 cc of normal saline in CT scanning room, and CT images were reconstructed with 3 mm slice thickness using a Philips Brilliance Big Bore CT scanner system (Philips Healthcare, the Netherlands).
Definitions for high- and intermediate-risk clinical target volume (HR-CTV/IR-CTV) are established in the IBS-GEC ESTRO-ABS recommendations [10], and relevant OARs included for IC/ISBT plans are the bladder, rectum, sigmoid, and small intestine. Treatment plan was created by reconstructing the applicator and needles, and optimizing dwell position and time using Oncentra treatment planning system (version 4.3; Nucletron, Elekta AB, Stockholm, Sweden).
Evaluation methods
To evaluate the accuracy of needle location, the variation of needle tip position was calculated from each interstitial implantation in BT plans, and defined the initial implantation as baseline. Coordinates of the placed needles were defined in reference to the same bony landmarks to maintain a uniform coordinate system for comparison. Deviation from the initial plan in terms of tip position were measured by image registration method using MIM software (version 7.1.6; Cleveland, USA), and CT images of all BT plans were reconstructed as 1 mm slice thickness for evaluation.
The requirement of IC/ISBT planning was that 90% of HR-CTV received 100% of prescribed dose (7 Gy per fraction), and 90% of IR-CTV received 5 Gy per fraction. Dosimetric parameter of dose volume histogram (DVH) included dose delivered to 90% of HR-CTV and IR-CTV, volume of HR-CTV receiving 150% and 200% of the prescribed dose (V150 and V200), conformal index (CI) of HR-CTV, and maximal dose delivered to at least 2 cc of the bladder, rectum, sigmoid, and small intestine (D2cc). Conformity index (CI) was defined in order to describe the quality of the target as follows:
where VT represents the volume of HR-CTV, VT.D90 is the target volume wrapped by reference D90 curve, and VD90 denotes the volume wrapped by reference D90 curve. A higher CI value, ranging from 0 to 1, represents better conformity.
Clinical evidence for dose effect is demonstrated for target, and local control rate is depending on tumor volume, according to the results from a multi-center patients’ series RetroEMBRACE [22]. The precision of applicator implantation will directly affect the dose distribution of target volume, and the implantation is in turn subject to the size of the tumor. Hence, the robustness of implantation methods should be evaluated through different tumor size and its dose levels. In this study, we used three geometric characteristics to define tumor size, including target volume, major axis, and eccentric distance. Major axis was defined as the maximum diameter of HR-CTV in transverse section, eccentric distance was defined as the maximum distance from the center to the edge of HR-CTV in the same CT slice. In order to verify the applicability and practicability of 3D standardized template for various target size, correlation analysis was applied to calculate the correlation coefficient between geometric characteristics and dosimetric metrics.
Statistical analysis
Dosimetric difference and variation of tip position between ST and FH groups were compared and analyzed using paired-sample t-tests, and p < 0.05 was considered statistically significant difference. Correlations between geometric parameters of HR-CTV and dosimetric parameters were evaluated with Spearman’s correlation analysis between the groups. All statistical analyses were performed using SPSS Statistics version 19.0 software (IBM, Chicago, IL, USA), and Python software (version 3.6.5, Anaconda Inc., USA).
Results
A total of 172 implants including 832 needles were performed as a part of definitive treatment for a total of 43 LACC patients (Table 1). The mean HR-CTV volume was 40.01 ±14.76 cc in the ST group, and 43.88 ±11.96 cc in the FH group (p = 0.348). The modalities of radiotherapy for all enrolled patients were volumetric-modulated arc therapy (VMAT) and iridium-192 high-dose-rate brachytherapy (HDR-BT). On average, the number of needles inserted per implant was similar for both the ST and FH groups (5.01 vs. 4.66, p = 0.335). Figure 2 shows the mean variation of tip position between the insertions for all needles of 1.41 ±0.70 mm (range, 0.3-3.0 mm) and 2.74 ±0.81 mm (range, 1.0-4.0 mm) in the ST and FH groups, with statistical significance (p < 0.001). Figure 3 indicates the needle placement reproducibility with the use of 3D standardized template.
Table 1
Clinical features of 43 LACC patients
SD – standard deviation, VMAT – volumetric-modulated arc therapy, HDR-BT – high-dose-rate brachytherapy
a Major axis is defined as the maximum diameter of HR-CTV in transverse section; b Eccentric distance is defined as the maximum distance from the center to the edge of HR-CTV in the same CT slice. Volume, major axis, and eccentric distance are geometric statistical parameters for HR-CTV in this work
Fig. 2
Box plot from comparing 3D standardized template with free-hand implantation for needle tip positioning variation in each BT plan. FH group is plotted in red, and ST group is in green
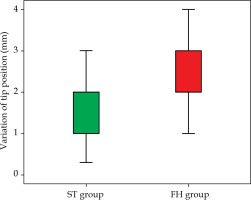
Fig. 3
Needle placement reproducibility between two fractional BT for a single patient. a1, b1, c1) Placement of needles for first BT in three CT slices; a2, b2, c2) placement of needles for second BT in three CT slices. a3, b3, c3) placement reproducibility of needles using images registration in three CT slices
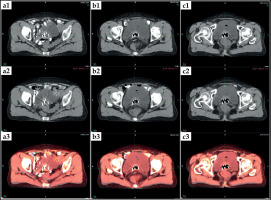
The comparisons of dosimetric metrics between the two groups using paired-sample t-tests are presented in Table 2. The ST group was superior in terms of CI, significantly improving 23.21% compared with the FH group (p < 0.001), which means that the dosimetric consistency was improved with the use of 3D standardized template. Furthermore, the D90 of HR-CTV and IR-CTV in the ST group were increased, 3.58% (p = 0.031) and 10.22% (p = 0.002) compared with the FH group. The D2cc of the bladder and sigmoid in the ST group were lower than those in the FH group (p < 0.05). The examples of dose distributions from 3D standardized template and free-hand implantation of IC/ISBT plans are illustrated in Figure 4.
Table 2
Dosimetric parameters of ST and FH groups in clinical BT plans
Fig. 4
Dose distribution of BT plans. a1-a6) A patient from ST group treated with 6 needles; b1-b6) a patient from FH group treated with 5 needles. Red solid and dashed lines represent HR-CTV in ST and FH groups, while blue represent IR-CTV. Colourwash represent dose distributions, ranging from 50% prescription to 100% prescription
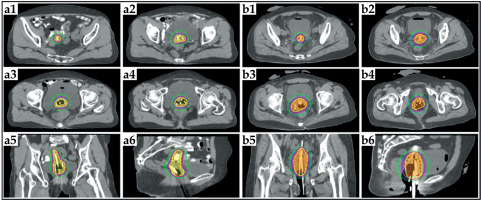
The results of Spearman’s correlation analysis between geometric parameters of HR-CTV and dosimetric parameters are presented in Table 3. The volume of HR-CTV and its CI in the ST group demonstrated strong positive correlation (R = 0.865, p < 0.001), while the major axis and eccentric distance showed moderate positive correlation (R = 0.554, p = 0.005, and R = 0.456, p = 0.025). However, the identical analysis revealed weak correlation in the FH group. These findings may demonstrate that even for larger tumors, 3D standardized template-guided IC/ISBT still have the potential for superior target dose coverage. The heatmaps of correlation analysis are illustrated for all of the correlation estimates in the two groups (Figure 5).
Table 3
Spearman’s correlation analysis between geometric parameters of HR-CTV and dosimetric para- meters
Fig. 5
Heatmaps of correlation analysis between dosimetric parameters and geometric parameters. A) Correlation analysis in ST group. B) Correlation analysis in FH group. Abscissa shows geometric parameters of HR-CTV, while ordinate demonstrates dosimetric parameters. Red denotes positive correlation, while blue is negative correlation. The darker the color, the greater the relevance
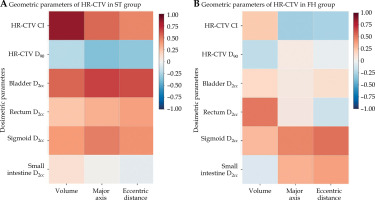
Discussion
The analysis from the RetroEMBRACE study [11] has demonstrated that IC/ISBT improved the therapeutic ratio in LACC by enabling tumor-specific dose escalation, resulting in significantly higher local control in large tumors without adding treatment-related late morbidity. The EMBRACE-I cohort study [12] also provided large-scale prospective clinical evidence of the feasibility and efficacy of IGBT in LACC treatment, with 43% of enrolled patients achieving 5-year local control rate of 92% using IC/ISBT. Although IC/ISBT technology has well-established dosimetric benefits and is associated with improved local control for patients with LACC, this technique requires greater technical skills than standard IC method [13]. In fact, more technical and manpower resources are required for modern IGBT procedures, with fewer reimbursement and higher fractional treatment time provided. Moreover, the addition of interstitial needles further increases the technical complexity of IGBT and likely involves a distinct learning curve for clinical use that may limit implementation of IC/ISBT among radiotherapy practitioners [14, 15]. Therefore, simplifying needle insertion and increasing treatment precision have the potential to be particularly beneficial in expanding the application of IC/ISBT technique as well as changing the technically challenging IGBT to one that is a component of routine practice. Moreover, decreasing the technical complexity of procedures may improve practitioners’ involvement for development of core BT skills.
Recently, several studies [7, 16, 17] have reported the use of 3D-printed templates for IC/ISBT, proposing patient-specific applicators, novel applicator designs, and optimized source trajectories. These prior published experiences tend to focus on using an individualized template for each patient with the lack of widespread clinical use of IC/ISBT technology. Marar et al. [8, 18] designed and developed a 3D-printed templates, named tandem anchored radially guiding interstitial templates (TARGITs) and next-generation TARGIT-Flexible-eXtended (TARGIT-FX), and achieved superior target coverage while sparing normal tissues. The primary purpose of the authors work was to simplify the needle insertion and increase the flexibility in needle placement. They proposed that the standard design of 3D-printed templates can be effectively applied for majority of patient cases in a way that is arguably more efficient that creating custom templates on a per-patient basis [18]. Therefore, the standardized flexible IC/IS template that is appropriate in a variety of tumor types can be the appropriate choice to promote the development of IC/ISBT technique.
In this study, the quick and accurate needle insertion using 3D standardized template allowed for minimal in-vasiveness that may reduce patient morbidity. The template-guided IC/ISBT presented better repeatability of needle tip position than free-hand implantation in fractional IGBT procedures (Figures 2 and 3). Meanwhile, the immobilization of applicators was easy using a bandage instead of placing gauze packing. Therefore, the precision, simplification, and convenience of using 3D standardized template have the potential to improve the confident implementation for IC/ISBT, especially among residents.
The superiority of dosimetry is one of the important factors to evaluate the performance of 3D-printed template-guided IC/ISBT [19, 20]. Our work also presents the comparisons of dosimetric metrics between the ST and FH groups. According to recommendations of the American Brachytherapy Society [21], the cumulative equivalent doses in 2 Gy (EQD2) of D90 for HR-CTV should exceed 80 Gy after EBRT and BT, while all patients in the current study met the requirements. The D90 of HR-CTV and IR-CTV in the ST group were increased to 3.58% (p = 0.031) and 10.22% (p = 0.002) compared with the FH group, while D2cc of the bladder and sigmoid were lower than those in the FH group (p < 0.05). These dosimetric results of 3D standardized template were similar to previous reports, in which individualized templates were mainly used [6-8, 16-20]. Furthermore, the mean value of CI in this study was significantly improved by 23.21% in the ST group compared with the FH group (p < 0.001). This was meaningful because of the superior dose conformality of the target using 3D standardized template. On the other hand, better conformality also indicated more precision and consistency of needle placement in every fractional IC/ISBT assisted with standardized template.
Apart from the evaluation of dosimetric differences, we used the correlation analysis to verify the adaptation of 3D standardized template (Table 3). The volume of HR-CTV and its CI in the ST group showed strong positive correlation (R = 0.865, p < 0.001), while the major axis and eccentric distance presented moderate positive correlation (R = 0.554, p = 0.005, and R = 0.456, p = 0.025). These findings may demonstrate that even for larger tumors, 3D standardized template-guided IC/ISBT still have the potential for superior target dose coverage without increasing normal tissue dose. Certainly, more needles would be generally arranged for larger tumors in both the groups. However, an identical analysis showed weak correlation in the FH group. Similar results were demonstrated by Liu et al., who showed dosimetric advantages for BT plans with larger target volume between 50 and 60 cc in LACC patients using 3D-printed template [23]. The correlation analysis emphasized that 3D standardized template was flexible and applicable to variety of tumor types, and achieved satisfied dosimetric characteristics.
To our knowledge, the present work is the first retrospective cohort study to evaluate the consistency and precision of 3D standardized template-guided IC/ISBT in LACC cases. However, there were some limitations, including small sample size and single center. Furthermore, this work did not compare dosimetric outcomes between 3D standardized template and commercially available IC or IC/IS applicators, as it was not the purpose of the study. Commercially available applicators are often expensive, require limitation with an afterloader device, and lack of adaptation for population and technical needs. On the other hand, 3D individualized templates are also out of our scope of comparison. Although customized templates present a solution to limitations of “one size-fits-all” commercial applicators, both IC/IS technique and 3D customized templates have a high learning curve that limits implementation in clinical practice. In addition, sub-group analysis of patients with large size HR-CTV, such as 30 cc, was absent in the discussion. The correlation analysis showed a weak link between HR-CTV D90 and volume in the ST and FH groups (Table 3), indicating that superior dose distribution can be achieved using IC/ISBT even for large target. Certainly, the clinical significance using standardized templates-guided IC/IS technique should be performed and verified by large clinical trials.
Conclusions
In summary, to facilitate a greater accessibility of IC/ISBT technique, we demonstrated that the 3D standardized template is an effective method of achieving improved precision and consistency in the needle insertion procedure at no cost of additional pre-plans (replace some customized 3D templates), and clinically satisfied dose distribution in IC/ISBT plans for patients with LACC. The flexibility, simplification, and adaptation approach of 3D standardized template can be incorporated within routine BT practices.