Purpose
Gynecological cancer is one of the most problematic malignancies worldwide. Cervical cancer is the most common gynecological cancer, and is the leading cause of death among women in many countries [1]. For endometrial and ovarian cancers, the mainstay of treatment is surgery. However, in cases with local recurrence after definitive treatment, brachytherapy serves as a salvage modality that can provide effective outcomes [2]. Vulvar cancer, though less common, is frequently observed in developing countries. Radiotherapy, including external beam radiation and brachytherapy, plays an important role in all stages of treatment, as does brachytherapy for vaginal cancer [3, 4].
Early-stage cervical cancer is cured by radical hysterectomy; however, isolated loco-regional recurrence has been reported in 5.6-38.8% of cases [5, 6]. High-dose-rate (HDR) brachytherapy is an appealing option for salvage treatment in cases with vaginal vault recurrence, particularly in patients who have previously undergone radiotherapy [7]. However, clinical outcomes and toxicities can vary among different cohorts. A systematic review of re-irradiation with 3D-IGABT (image-guided adaptive brachytherapy) in recurrent gynecological cancers reported a 2-year local control of 50-65.8% and a 2-year overall survival (OS) rate of 61-78%. In terms of toxicity profiles, grade 3 toxicity was reported in 4.5-17% of cases, with one series reporting a grade 4 fistula rate of 11.1% [8].
Vaginal recurrence is one of the most common sites of relapse after definitive treatment of endometrial cancer [9, 10]. Brachytherapy is generally used as a salvage treatment for local recurrence, and can contribute to a high-rate of local control with acceptable toxicity [2]. A study by Ling et al. reported the outcomes of re-irradiation with brachytherapy for vaginal recurrence of endometrial cancer, and showed a local control rate of 65.8%, with no grade ≥ 3 acute or late gastrointestinal (GI) and genitourinary (GU) toxicities [11]. Another single-institution cohort of 37 patients demonstrated the outcomes of interstitial brachytherapy as salvage treatment for recurrent endometrial cancer, with 4-year OS, local control (LC), and progression-free survival (PFS) rates of 81.0%, 77.9%, and 56.8%, respectively. Most patients experienced grade 1-2 adverse events, and no grade 3-4 toxicity was observed [12].
For vulvar cancer, several studies have shown a trend towards improved complete response rates with escalated-dose concurrent chemoradiation [3, 13, 14]. One way to increase the dose is using brachytherapy in combination with external beam radiation therapy (EBRT). A study of combined treatment, EBRT and HDR brachytherapy with a median value of 72.4 Gy EQD2, showed favorable loco-regional control. However, some grade 3 toxicities were observed, such as vaginal pain (17.7%) and skin/vaginal necrosis (5.9%) [15].
Despite advances in treatment with EBRT and brachytherapy, there are challenging cases, where the desired dose to high-risk clinical target volume (HR-CTV) cannot be achieved. A 3D printing method in combination with intra-cavitary or interstitial procedure was adopted to improve the target coverage in advanced cases, wherein conventional applicators were unsuitable. Several studies have highlighted the benefit of applying 3D printing techniques in brachytherapy. For example, Logar et al. tested 3D-printed applicators in nine patients with advanced gynecological cancers, demonstrating that all dose-volume histogram (DVH) parameters for HR-CTV significantly improved, without compromising dose constraints for organs at risk (OARs) [16]. Another study investigated the clinical outcomes of using 3D-printed applicators for vaginal cancer recurrences in 26 patients treated with a combination of EBRT and brachytherapy, with a mean dose of 71 Gy EQD2. The 2-year local control rate was 87.8%, with minimal late grade 3 toxicity (4%) [17].
This latter study emphasizes the importance of personalized brachytherapy needle applicators developed using 3D printing technology. The 3D-printed template featured 8 holes to facilitate precise insertion of the needles parallel to tandem cylinder. The results demonstrated exceptional coverage of the target, confirming its efficacy and potential for improving brachytherapy outcomes [18]. Another study from the EMBRACE group reported the clinical outcomes of using 3D-printed vaginal tandem-needle templates for trans-vaginal insertion of needles in parallel and/or oblique direction in 19 patients. Most patients were stage III-IV, with a mean D90 HR-CTV of 93 Gy (EQD2). The 3-year local control rate was 95%, with grade ≥ 3 treatment-related complications in only 10% of patients. This study supports the role of 3D-printed applicators in patients with extensive local disease or unfavorable anatomy [19].
While the clinical outcomes of using 3D printing in brachytherapy have primarily been reported for cervical cancer [19], there are several challenging gynecological cancer cases, where 3D printing technology can play an important role when considering brachytherapy. Therefore, the current study aimed to evaluate the tumor local control and treatment-related toxicity in gynecological cancer patients treated with 3D printing-based image-guided adaptive brachytherapy.
Material and methods
Data of patients with both primary and recurrent gynecological cancers treated with in-house 3D-printed applicators at Siriraj Hospital during 2021-2022 were retrospectively reviewed. The study was approved by the Institutional Ethics Committee (Si 553/2022).
Patients treated with 3D printing technique were those deemed unsuitable for treatment with available commercial applicators, as determined by their physicians. These cases were then discussed at the tumor board to assess the necessity of using 3D printing technique. The criteria for deciding on the use of 3D printing were based on the following:
Criteria 1: Patients with highly advanced diseases
Patients considered for 3D-printed applicators were those with highly advanced diseases (for example, stage IIIB or stage IVA cervical cancer), who could not be adequately covered with standard applicators, including interstitial implantation. While free-hand interstitial implantation may help improve D90 of HR-CTV to achieve clinical goal, the reproducibility of implantation depends on the radiation oncologist. In contrast, 3D printing enables higher reproducibility for each fraction due to the rigid channels for needle insertion. Although various commercial needle templates exist, none were available at our institute.
Criteria 2: Patients, for whom standard applicators could not be fitted
In cases, where standard applicators cannot be applied, 3D printing allows customizing of the applicator to fit each patient’s anatomy. In cases with a narrow vaginal canal, one-size-fits-all applicators may not be insertable, possibly due to previous radiation and/or brachytherapy. In such cases, 3D printing increases the opportunity to perform brachytherapy in all patients, regardless of the anatomy.
In the current study, the 3D printing process started with a pre-planning step, where the patient was examined by a physician, and vaginal/vulvar gauze packing was performed with or without placing the markers. CT and/or MRI simulation was done, and images were transferred to treatment planning system. Contouring was accomplished based on the images and markers. For dose planning process, the physicist created the presumed paths of needles to cover the targets. Coordinates of the paths were transferred to 3D printing software, and shapes of the applicators were created based on the packing. After the models were printed, the final step involved smoothing the surface of applicators. The patient was scheduled to undergo brachytherapy procedure 2 weeks after the pre-planning day. The process of producing and the examples of 3D-printed applicators are shown in Figures 1 and 2, respectively.
Fig. 2
Examples of reconstructing 3D-printed applicators. A) The model with channels before smoothing the surface, B) the model after smoothing the surface, and C) printed version of the model
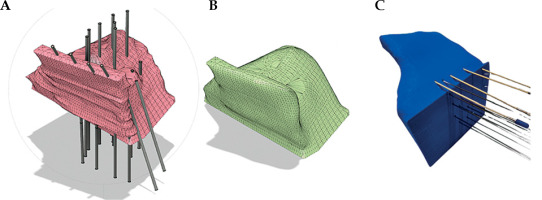
During the procedure, patients were given sedation and pain control, such as midazolam and fentanyl. The 3D-printed applicators were applied by radiation oncologists, followed by insertion of needles using trans-rectal ultrasonography guidance if needed. Patients who were treated with 3D-printed applicators were prescribed a radiation dose similar to that given with standard applicators. For cervical cancer patients, definite concurrent chemoradiation with external beam radiation (EBRT) of 45-50 Gy in 25 fractions and HDR brachytherapy in 4 fractions (7-8 Gy/fraction) was administered. For vulvar cancer patients, EBRT of 45-50 Gy in 25 fractions and HDR brachytherapy in 4 fractions (5-6 Gy/fraction) or brachy-monotherapy of 5-6 Gy in 6-10 fractions was administered. For cases with vaginal recurrence, EBRT of 45-50 Gy in 25 fractions and HDR brachytherapy in 4 fractions (7-8 Gy/fraction) or brachy-monotherapy of 6-7 Gy for 6-8 fractions was administered. The examples of 3D-printed applicators from both Criteria 1 and Criteria 2 with dose distribution are shown in Figure 3.
Fig. 3
A) Example of 3D-printed applicator according to Criteria 1, with CT simulation images showing 100% isodose line. B) Example of 3D-printed applicator according to Criteria 2, with the application and immobilization process. C) CT simulation images showing needle reconstruction and 100% isodose line
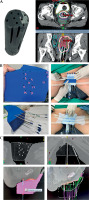
Staging for each malignancy was based on the FIGO 2018 classification. The timing and pattern of response after treatment were reported. Local relapse-free survival (LRFS) was defined as the time from the start of treatment to local recurrence. Distant metastasis-free survival (DMFS) was described as the time from the start of treatment to distant metastasis. Progression-free survival was defined as the time from the start of treatment to any progression, either from local recurrence, regional recurrence, or distant metastasis. Overall survival was specified as the time from the start of treatment to death, regardless of disease recurrence. At 3 months, the treatment response was evaluated based on the response evaluation criteria in solid tumors (RECIST). Complete response (CR) was defined as no residual tumor based on clinical examination and radiographic imaging, either by CT or MRI. Partial response (PR) was described as at least a 30% decrease in the sum of the longest diameter (LD) of target lesions, using the baseline sum of LD as a reference.
Kaplan-Meier estimates at 1 year after treatment were analyzed for local recurrence-free survival, distant metastasis-free survival, progression-free survival, and overall survival. The actuarial rates of acute and late GU, GI, and skin and mucosa toxicities were graded according to CTCAE version 5.0. This study was approved by the Institutional Review Board.
Results
The median follow-up time was 27 (IQR: 15.4-32.5) months. Among the 14 patients, 5 patients (35.7%) were categorized with primary and 9 patients (64.3%) with recurrent disease. Among the primary cases, 2 patients had cervical cancer, and 3 patients had extra-mammary Paget’s disease of the vulva. Among the recurrent cases, all patients had vaginal relapse, and the most common primary cancers were cervical cancer (4 patients, 44.4%) and ovarian cancer (3 patients, 33.3%). There was one case (7.1%) with recurrence from primary endometrial cancer, and one patient with recurrent high-grade squamous intra-epithelial lesion (HSIL) of the vagina. In terms of radiation technique, the majority of patients (9 patients, 64.3%) were treated with brachytherapy alone, while 5 patients (35.7%) received EBRT in combination with brachytherapy. The brachytherapy techniques most used in this study were the intra-cavitary and mold technique. Patients with a vulvar lesion were generally treated with the mold technique, while patients with a recurrence at the vaginal stump were treated with the interstitial technique. The median number of reconstructed channels used per 3D-printed applicator was 7 channels. The patients baseline characteristics are presented in Table 1.
Table 1
Baseline patient characteristics
Among the entire cohort, 6 patients (42.8%) were selected for 3D printing based on Criteria 1, and 8 patients (57.2%) were chosen based on Criteria 2. The mean dose of D90 HR-CTV for patients who received EBRT followed by brachytherapy was 85.3 Gy (EQD2), and 49.3 Gy (EQD2) for brachytherapy alone. The details of the prescribed dose fractionation and received doses for each patient are shown in Table 2. At 3 months post-treatment, 13 patients (92.9%) achieved CR and 1 patient (7.1%) PR. A swimmer plot showing the treatment response and pattern of recurrence for each patient is illustrated in Figure 4.
Table 2
Dose fractionation, number of needle channels, and D90 HR-CTV of each patient
Among primary cases, no patient developed recurrence or distant metastasis, nor death occurred. The rates of 2-year LRFS, DMFS, PFS, and OS were all 100%. For recurrent cases, the 2-year LRFS was 74.07%. Two patients developed local recurrence after completion of treatment, and both the patients had in-field recurrence at the same site as the initial tumor. The time from the completion of salvage brachytherapy to second local recurrence was 3 and 19 months, respectively. The 2-year DMFS rate was 88.9%. One patient with recurrent ovarian cancer in the vagina and another patient with recurrent cervical cancer in the vagina developed distant metastases, and the sites of metastases included the lung, mediastinal lymph node, and bone. The time from the completion of salvage brachytherapy to distant metastasis was 5 and 36 months for recurrent ovarian cancer and recurrent cervical cancer, respectively. The rates of 2-year PFS and OS were 64.8% and 88.9%, respectively. There were two deaths observed at 12.4 months and 36.5 months after completion of salvage brachytherapy. Kaplan-Meier curves for LRFS, DMFS, PFS, and OS are demonstrated in Figure 5.
Fig. 5
Kaplan-Meier curves for A) local relapse-free survival, B) distant metastasis-free survival, C) progression-free survival, and D) overall survival
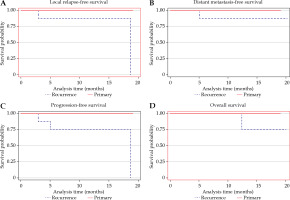
The actuarial rates of ≥ grade 2 acute GU and GI toxicities were 7.1% for both. The most common acute urinary symptoms were increased frequency and dysuria, while the most common acute gastrointestinal symptom was diarrhea. For ≥ grade 2 late GU and GI toxicities, the actuarial rates were 14.3% and 21.4%, respectively. No grade 3 GU and GI toxicities were observed in this study. The most common late grade 2 urinary symptom was urinary tract obstruction due to ureteric stricture, while the most common late gastrointestinal symptom was proctitis. In terms of skin and mucosa toxicities, 4 patients (28.6%) developed acute grade 3 toxicity; however, the symptoms subsided within 3 months after the completion of treatment. One patient (7.1%) had persistent grade 3 vaginal toxicity with vaginal stenosis. The mean of maximum skin and vagina dose among patients receiving brachytherapy at vulvar and vagina sites was 141.3 ±33.3 Gy and 154 ±18 Gy, respectively. The patient with persistent grade 3 vaginal toxicity received the maximum vaginal dose of 172 Gy.
Comparing the toxicity between patients who received brachytherapy alone versus those who received both EBRT and brachytherapy, one patient in each cohort developed grade 2 GU and GI toxicities, with no statistically significant difference (p = 0.37). For skin and mucosal toxicities, all patients with grade 3 toxicity received brachytherapy alone. No patients in the EBRT plus brachytherapy group developed grade 3 skin and mucosal toxicities; however, the difference was not statistically significant (p = 0.19).
Discussion
Given the complexities of various gynecological cancers and their anatomical variations, a standardized one-size-fits-all approach to brachytherapy applicators might not be optimal. This has prompted the development of 3D-printed applicators, tailored to each individual patient’s anatomy, to enhance target coverage in advanced cases, where conventional applicators are insufficient. We proposed the following criteria for patient selection for a 3D-printed applicator: First, patients with highly advanced disease, for whom conventional applicators cannot provide adequate coverage; Second, patients, for whom standard applicators do not fit due to anatomical variations. These criteria were based on challenges encountered with difficult cases. Even though patients with advanced diseases can be treated with free-hand implants or commercial templates, 3D-printed applicators offer an advantage in terms of higher reproducibility across each fraction and reduced procedure time. For patients not suited for standard applicators, 3D-printed applicators provided greater benefits, as they otherwise would not be able to undergo brachytherapy treatment.
Previous studies have shown the benefits of 3D-printed applicators in improving dose distribution, with a minimal impact on organ at risk constraints [17]. Moreover, 3D-printed applicators can also facilitate the insertion procedure, which was observed in this study. A single-institution retrospective study analyzing a cohort of 148 patients treated with a 3D-printed tandem anchored radially guided interstitial template (TARGIT) reported that it can increase the simplicity of insertion techniques for tandem and ovoid applicators. The result revealed that 3D-printed applicators reduced the procedure time, while enabling a 30% increased tumor coverage and similar normal tissue sparing compared with conventional applicators [20].
The current study had significant benefits, showing a high tumor response with a CR rate of 92.9% and a PR rate of 7.1% at 3 months post-brachytherapy. The 2-year local relapse-free survival rate for recurrent cases in this study was 74.07%, similar to the results of patients with recurrent vaginal stump from endometrial cancer treated with salvage HDR brachytherapy using standard applicators, who achieved a 3-year local control rate of 65.8% [11]. For primary cases, this study reported a 2-year local control rate of 100%. Comparable outcomes were observed by Cobussen et al., whose study included 101 patients treated with 3D-printed vaginal tandem-needle templates, reaching a 3-year local control rate of 85% [19]. Despite similar local control rates, the patients in Cobussen’s study were suitable for standard applicators, whereas the patients in the current study were not suitable for standard applicators [7]. The comparison of clinical outcomes among the afore-mentioned studies is shown in Table 3.
Table 3
Comparison of clinical outcomes using 3D-printed applicators for brachytherapy in the present study and the literature
Parameter | Cobussen et al.[19] | Qin et al. [17] | Present study |
---|---|---|---|
Patients | 19 cervical cancer patients | 26 patients with central pelvic- recurrent cervical cancer | 14 patients with gynecological cancer, both primary and recurrent |
Applicators | 3D-printed vaginal tandem-needle templates | Various customized 3D-printed applicators | Various customized 3D-printed applicators |
Local control | 3-year LC: 85% | 2-year LC: 87.8% | 2-year LRFS: 74.07% |
Acute toxicity | N.A. | Grade 3-4 hematological toxicity: 21% No grade 3-5 acute GU or GI toxicities | ≥ grade 2 GU: 7.1% (no grade 3) ≥ grade 2 GI: 7.1% (no grade 3) Grade 3 skin and mucosal toxicities: 28.6% |
Late toxicity | ≥ grade 3 treatment-related complications: 10% | Grade 3 GI (recto-vaginal fistula): 4% | ≥ grade 2 GU: 14.3% ≥ grade 2 GI: 21.4% Grade 3 vaginal stenosis: 7.1% |
In terms of toxicity profiles, this study presented acceptable rates of acute GU and GI toxicities, with actuarial rates of ≥ grade 2 being 7.1% for both. However, the rate of late toxicity was higher for GI toxicity at 21.4% compared with 14.3% for late GU toxicity. These rates are comparable to those reported in another study on HDR brachytherapy for salvage recurrence using 3D printing techniques [2, 11, 12]. However, treatment-related non-GU/GI toxicities were reported at relatively higher rates compared with GU and GI toxicities. Qin et al. reported acute grade 3-4 hematological toxicity as 21% [17]; however, hematological toxicity was not recorded in this study or in a comparative research. In the present study, acute grade 3 skin and mucosal toxicities were reported in 28.6% of patients, commonly observed in those treated with vaginal and vulvar brachytherapy. We also observed that patients with persistent late vaginal toxicity tended to obtain the highest maximum vaginal dose. Other treatment-related adverse events from 3D-printed brachytherapy should be further investigated.
The key material used in this study was PLA filament, because it is processed from non-toxic plant components, which are safe for the environment, and the cost per unit of the filament was inexpensive. Moreover, the accuracy of dose absorption and distribution in PLA materials was reported using a gamma method (3%, 3 mm) at 1 posterior and 2 superior side (0 and 0.5 cm apart), and passing rates of each position were found to be 100%, 98.56%, and 99.01% for the multi-channel, and 98.6%, 99.13%, and 99.45% for the ring with a single-channel, which showed enough robustness for clinical use [21].
The overall cost of producing one 3D-printed applicator was remarkably low compared with commercial applicators. The average cost to produce a single 3D-printed applicator was $40, covering all expenses for the entire process. The total time required, including pre-planning and manufacturing, averaged 2 weeks, with a typical printing time of 8 hours per applicator. This suggests a production capacity of 30 applicators per month. Currently, only 5 patients per month require 3D-printed applicators, leaving additional capacity in our department to expand this service in the future. However, on the other side, the in-house 3D-printed applicators were aimed to be used for one patient, while commercial applicators can be re-sterilized and used multiple times. Therefore, a cost-effectiveness analysis comparing 3D-printed and commercial applicators should be further conducted, in order to help clinicians to determine when and for whom the 3D-printed applicator technique should be adopted as a sufficient option.
Tailoring applicators to individual patient’s anatomy improve target coverage and potential outcomes, especially in challenging cases. However, the manufacture of 3D-printed applicators is usually time-consuming. Future development should focus on universal applicators for individuals with unique anatomy or specific sites, with reduced procedure time. For example, the Siriraj ring cap was developed for patients with large tumors, which needed a needle implant [22]. While most studies have focused on cervical cancer, the effects of this study concern other gynecological malignancies, such as the vulva and vagina.
Despite the benefits of the study, there are several limitation to be acknowledged, including a retrospective analysis, small sample size, short follow-up period, and lack of direct comparison.