Purpose
Prostate cancer is one of the most common malignancies in men and is a major cause of cancer death [1]. Prostate-specific antigen (PSA) screening enables the detection of early and localized prostate cancer. There are many therapeutic options for patients with localized prostate cancer, including androgen deprivation therapy (ADT), radical prostatectomy, external beam radiation therapy, and brachytherapy. Permanent seed brachytherapy, also termed low-dose-rate (LDR) brachytherapy, enables a high-dose of radiation to the prostate, with a steep dose gradient to the surrounding normal tissues. Previous reviews have shown that outcomes of brachytherapy for localized prostate cancer are comparable to those of other therapeutic modalities, including radical prostatectomy and external beam radiation therapy [2,3,4].
Zauls et al. first reported intraoperatively built custom-linked (IBCL) seeds, as a push-button delivery system that uses a combination of seeds, connectors, and spacers [5]. LDR brachytherapy was first adopted in 2003 in Japan, but stranded or linked seeds were not introduced until 2012, and only loose seeds (LS) had been used for LDR brachytherapy until then [6]. Several studies have reported the advantages and disadvantages of IBCL seeds compared with LS [5,7,8,9,10,11,12,13]. IBCL seeds have the advantages of less migration, and stability due to intraoperative linking, indicating the benefits of loose and stranded seeds [14]. Therefore, we hypothesized that compared with LS, the use of IBCL seeds might reduce variance in the dosimetric parameters. To the best of our knowledge, no previous report has evaluated the variability in dosimetric parameters in a comparison of IBCL seeds and LS.
Moreover, the optimal timing for obtaining post-implant computed tomography (CT) and/or magnetic resonance (MR) imaging remains a debated issue. Because prostatic edema arises in the first 24 hours after implantation, post-implant imaging obtained within 24 hours results in lower calculated doses [15,16]. Some studies suggest an interval of 2 to 6 weeks after implantation, when prostatic edema has decreased [17,18]; whereas others have recommended dosimetric evaluation within 24 hours, because this allows for immediate correction of a possible dose deficiency [19,20,21]. In addition, although most previous reports refer to the dosimetric parameters of the whole prostate, some have evaluated dose distribution in different regions of the prostate [10]. We have previously reported the usefulness of sector analysis for localized prostate cancer treated with LS [22].
The purpose of this study was to compare the dose distribution and to evaluate the variation in dosimetric parameters between IBCL seeds and LS, using sector analysis at 24 hours and 1 month after brachytherapy.
Material and methods
Patient characteristics
This study was approved by our Institutional Review Board and was conducted in accordance with the principles of the Declaration of Helsinki. Patients treated with brachytherapy alone at our institution were eligible for this study. A total of 64 consecutive patients were retrospectively analyzed, including those treated with LS between March 2013 and August 2015 (LS group, n = 32), and those treated with IBCL seeds between September 2015 and October 2017 (IBCL group, n = 32). Patients were staged according to the American Joint Committee on Cancer guidelines (7th edition), and were classified into prognostic risk groups according to the National Comprehensive Cancer Network (NCCN: www.nccn.org) guidelines. Neoadjuvant ADT to reduce prostate volume was generally administered to patients, with volumes > 40 cc.
Pre-implant planning
MRI-based pre-implant volume evaluations were performed, with the patient in the supine position, using a 1.5 T Avanto scanner (Siemens Medical Solutions, Erlangen, Germany) at a median of 8 weeks (range, 2-20 weeks) before implantation. T2-weighted 3D MR images of 2.5 mm thickness were imported into a treatment planning system (TPS). The dosimetry of pre-implant planning aimed for a prostate V100 (PV100, the percentage of the volume receiving 100% of the prescribed dose) of > 95%, a prostate D90 (PD90, the minimum dose received by 90% of the volume) of > 110% and < 130% of the prescribed dose, a prostate V150 (PV150, the percentage of the volume receiving 150% of the prescribed dose) of < 60%, a urethral D30 (UD30, the minimum dose received by 30% of the volume) of < 150%, and a rectal V100 (RV100, the volume receiving 100% of the prescribed dose) of < 0.2 cc. Urethral V150 (UV150) was also recorded, but not used as a dose constraint.
Implant procedure
The implant procedure was performed under spinal anesthesia, with the patient in the dorsal lithotomy position. For all patients, iodine-125 seeds (BARD, BrachySource model, Covington, GA, USA) with an apparent activity of 0.342 mCi and an air kerma strength of 0.432 U (μGym2/h) were used, and the prescribed brachytherapy dose was 145 Gy. The seeds were implanted transperineally with a Mick applicator (Mick Radio-Nuclear Instruments, New York, NY, USA) and under the guidance of real-time transrectal ultrasonography. A modified peripheral loading technique was used to deliver the dose to the prostate, avoiding placement of seeds close to the urethra [23]. Dose-volume constraints for the prostate, urethra, and rectum were the same as in the pre-implant planning. Until March 2014, an Interplant (Computerized Medical Systems, Champaign, IL, USA) TPS was used for pre-implant and real-time planning, and an Oncentra (Nucletron, Veenendaal, The Netherlands) TPS was used from April 2014.
Post-implant dosimetry
Post-implant axial CT images of the pelvis were obtained 24 hours and 1 month (median, 29 days) after the implant procedure, using an Asteion (Toshiba Medical Systems, Tokyo, Japan) at 2.0 mm thickness with the patient in the supine position. Post-implant dosimetric analysis was re-planned with the Oncentra TPS by a single radiation oncologist (E.O.). CT-MR image fusion for delineation was performed for all patients with the Oncentra TPS, using the MR images obtained in the pre-implant volume studies. The percentage changes in the volumetric parameters after 24 hours and 1 month were defined as follows:
Homogeneity index (HI) was defined as follows [24]:
The prostate volume was divided into four quadrants, such as anterior-superior quadrant (ASQ), posterior-superior quadrant (PSQ), anterior-inferior quadrant (AIQ), and posterior-inferior quadrant (PIQ). The superior- inferior and anterior-posterior segments were divided by the midpoint of the prostate on reconstructed CT images (Figure 1) [22].
Fig. 1
Division of the prostate into four quadrants
ASQ – anterior-superior quadrant, PSQ – posterior-superior quadrant, AIQ – anterior-inferior quadrant, PIQ – posterior-inferior quadrant
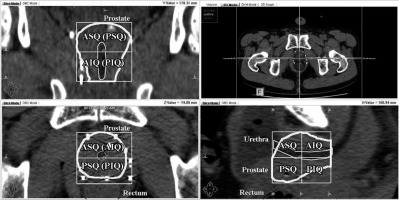
Seed migration was defined as the presence of one or more seeds ≥ 2 cm outside the prostate contour on a post-implant pelvic CT or X-ray. Seed loss was defined as unintentional reduction of seed number at the post-implant evaluation, excluding migrated seeds.
Patient follow-up
The date of implantation was considered day 0 in the analysis of follow-up duration. Patients were assessed every 2-3 months during the first to third year, and every 3-6 months at 3-10 years after brachytherapy. Serum PSA levels and adverse events data were collected at the clinical follow-up visits. Biochemical recurrence was determined according to the Phoenix definition [25]. Genitourinary (GU) and gastrointestinal (GI) adverse events were documented using the Common Terminology Criteria for Adverse Events, version 4.0.
Statistical analyses
An F-test was applied to compare the variance of the two groups, prior to t-test. T-tests (p ≥ 0.05 in F-test) or Welch’s t-test (p < 0.05 in F-test) were used for continuous variables when comparing the baseline characteristics in the two groups, and Fisher exact test was used for categorical variables. One-way analysis of variance (ANOVA) with Tukey’s honest significant difference (HSD) was applied for post-hoc testing. Coordinate points of a receiver operating characteristic (ROC) curve were used to identify significant dosimetric parameters for predicting adverse events. Statistical analyses were performed using R environment (version 3.2.2) available from http://www.R-project.org. A p value of < 0.05 was considered significant.
Results
Table 1 lists the patient characteristics. The median follow-up time was 49.9 months in the LS group and 27.1 months in the IBCL group (p < 0.001). Fourteen patients received neoadjuvant ADT 3-6 months before brachytherapy (luteinizing hormone-releasing hormone agonist, n = 10; maximum androgen blockade [combination of luteinizing hormone-releasing hormone agonist and anti-androgen], n = 2; anti-androgen, n = 2). No biochemical recurrence occurred during the follow-up period, and one patient died of lung cancer.
Table 1
Patient characteristics (n = 64)
Dosimetric analysis
Seed migration occurred in 11 patients (15 seeds: pelvis, n = 6; seminal vesicle, n = 5; lung, n = 4) in the LS group and in 3 patients (4 seeds: seminal vesicle, n = 3; perineum, n = 1) in the IBCL group (p = 0.032). Seed loss (excretion via urethra) occurred in 8 patients (14 seeds) in the LS group and none in the IBCL group (p = 0.005).
Table 2 presents the post-implant dosimetric data. PV150, UV150, and UD30 at 24 hours and UD30 at 1 month were significant by F-test, and standard deviation (SD) tended to be lower in the IBCL group than in the LS group (9.2 vs. 13.2, 5.5 vs. 17.6, and 11.7 vs. 18.5 at 24 hours, and 14.0 vs. 24.3 at 1 month, respectively), and similar trends were seen for most other dosimetric variables except PV100 at 24 hours. Analysis of the variables revealed significant difference for ΔPV100 and ΔPD90 by F-test, ΔPV150 and ΔUD30 showed marginal statistical significance, and the SDs of all variables were lower in the IBCL group. PV150, UV150, and UD30 at 24 hours and 1 month were significantly lower in the IBCL group compared with the LS group (t-test). There was no significant difference between the two groups in terms of PV100, PD90, and RV100 at 24 hours and 1 month. HI at 24 hours and 1 month was significantly higher in the IBCL group compared with the LS group (both p < 0.001).
Table 2
Post-implant dosimetric data for the prostate, urethra, and rectum (24 hours and 1 month)
* T-test was performed for variables with p ≥ 0.05 in F-test, and Welch’s t-test was performed for variables with p < 0.05, V100 – the percentage of the volume receiving 100% of the prescribed dose, V150 – the percentage of the volume receiving 150% of the prescribed dose, D90 – the minimum dose received by 90% of the volume, D30 – the minimum dose received by 30% of the volume, HI – homogeneity index, LS – loose seed, IBCL – intraoperatively built custom-linked
Table 3 shows the results of dosimetric analysis for each quadrant of the prostate. In the analysis of change in the variables, SD of ΔD90, PSQ, AIQ, and PIQ was significantly lower in the IBCL group than in the LS group (F-test; p = 0.018, < 0.001, and < 0.001, respectively). At 24 hours, D90 of PIQ was significantly lower in the LS group than in the IBCL group (p = 0.006), and D90 of ASQ was significantly higher in the LS group than in the IBCL group (p = 0.037). At 1 month, there was no significant difference between the two groups in terms of D90 in any quadrant. In comparison of the quadrants using one-way ANOVA with Tukey’s HSD, in the LS group, D90 at 24 hours was significantly lower in PIQ than in the other quadrants, and D90 at 1 month was significantly higher in AIQ than in the other quadrants. In the IBCL group, there was no significant difference in D90 at 24 hours among the quadrants, and D90 at 1 month was significantly higher in AIQ than in the other quadrants. V150 was significantly or almost significantly lower in the IBCL group compared with the LS group in almost all quadrants, except PIQ at 24 hours.
Table 3
Post-implant dosimetric data for the four quadrants of the prostate (24 hours and 1 month)
* T-test was performed for variables with p ≥ 0.05 in F-test, and Welch’s t-test was performed for variables with p < 0.05, ASQ – anterior-superior quadrant, PSQ – posterior-superior quadrant, AIQ – anterior-inferior quadrant, PIQ – posterior-inferior quadrant, V100 – the percentage of the volume receiving 100% of the prescribed dose, V150 – the percentage of the volume receiving 150% of the prescribed dose, D90 – the minimum dose received by 90% of the volume, LS – loose seed, IBCL – intraoperatively built custom-linked
To analyze the learning curve of LDR brachytherapy, patients from each group were divided into subgroups of 10, 10, and 12 patients, according to the date of implantation. The learning curve was analyzed using PD90, PV100, and PV150, and no significant difference was observed in terms of the treatment period in each group.
Analysis of adverse events
No grade 3 or higher GU or GI adverse events were observed. Two patients (6.3%) in each group experienced at least one episode of hematuria. Two patients (6.3%) in the LS group and 1 patient (3.1%) in the IBCL group experienced rectal hemorrhage. Figure 2 shows the frequency of grade 2 GU adverse events between 3 and 12 months after brachytherapy. At 3 months after treatment, the frequency of GU adverse events was significantly lower in the IBCL group (p = 0.032), and there was no significant difference in the other periods. Using UV150 and UD30 at 1 month, ROC analysis was performed for the frequency of GU adverse events after 3 months. The respective cut-off values for UV150 and UD30 at 1 month were 43.5% (AUC = 0.70, sensitivity = 66.7%, specificity = 74.4%) and 162.1% (AUC = 0.71, sensitivity = 66.7%, specificity = 76.7%). Figure 3 shows the change in the frequency of grade 2 GU adverse events using the cut-off value of UV150; significant differences at 3, 6, and 9 months (p < 0.001, 0.019, and 0.044, respectively) were noted.
Fig. 2
Incidence of grade 2 or higher GU adverse events between 3 months and 1 year after brachytherapy in the LS and IBCL groups
*Statistically significant difference between the two groups, LS – loose seed, IBCL – intraoperatively built custom-linked, GU – genitourinary
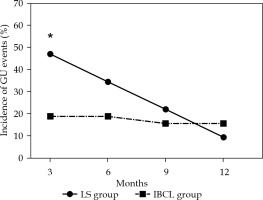
Fig. 3
Incidence of grade 2 or higher GU adverse events between 3 months and 1 year after brachytherapy, based on a cut-off value of UV150
*Statistically significant difference between the two groups, UV150 – urethral V150 (the percentage of the volume receiving 150% of the prescribed dose), GU – genitourinary
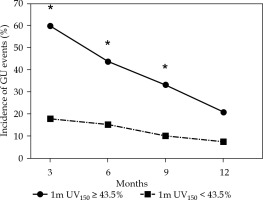
Discussion
The results of this study show that compared with LS, the SD of most dosimetric variables was lower for IBCL seeds, and statistically significant differences between the two groups were found for several variables (F-test; PV150, UV150, and UD30 at 24 hours, and UD30 at 1 month). Although sector analysis of V150 and D90 showed a general decrease in the SD for all sectors in the IBCL group, no statistically significant difference was observed. Analysis of changes in the variables showed a significant or near-significant decrease in SD; in sector analysis, the SD of changes in D90 in the IBCL group was also significantly lower compared with the LS group. These results indicate that compared with LS, the use of IBCL seeds can reduce variance of the dosimetric parameters. More importantly, the reduction in variance due to the use of IBCL seeds was more pronounced in the analysis of changes between 24 hours and 1 month, which indicates that compared with LS, using IBCL seeds improves the accuracy of predicting dosimetric parameters at 1 month, using those obtained at 24 hours. Furthermore, this result may ensure the validity of early dose correction, when dose deficiency is recognized as early as 24 hours after brachytherapy, when IBCL seeds are used.
The use of IBCL seeds also makes it possible to reduce high-dose areas in the prostate, improve HI, and reduce urethral dose for the whole prostate as well as each sector. PV100 and PD90 were not significantly different between the two groups. Many studies have compared dosimetric parameters between these groups, but the results are controversial (Table 4). Several papers have reported no significant difference in PV100 and PD90 between the two groups [5,8,10]. Inada et al. reported that PV100 was significantly higher in the IBCL group than in the LS group, whereas other studies showed that PD90 was significantly lower in the IBCL group than in the LS group [7,11,12,13]. In several studies, PV150 and UD30 were significantly lower and HI was significantly higher in the IBCL group than in the LS group, consistent with the results of the present study [7,11]. Two studies have reported that RV100 was significantly lower in the IBCL group than in the LS group, but we found no significant difference in the present study [7,13]. We consider that the decrease in variation of dosimetric parameters and the reduction of high-dose areas in both the prostate and urethra are due to the following characteristics of IBCL seeds compared with LS: (i) reduction of seed migration and loss, (ii) suppression of unintentional movement within the prostate after seed implantation, (iii) correct distance between seeds due to use of spacers, and (iv) the ability to intentionally place the seeds outside the prostate, which is particularly useful in peripheral loading techniques, and contributes to reducing urethral dose.
Table 4
Previously reported data of dosimetric comparisons between LS and IBCL seeds
Study | Year | N | Dose | Parameters | LS | IBCL | p-value |
---|---|---|---|---|---|---|---|
Zauls et al. [5] | 2010 | 91 | 100 Gy (Pd) | PD90 (Gy) | 98.2 | 104.0 | 0.42 |
160 Gy (I) | 162.7 | 164.9 | 0.36 | ||||
100 Gy (Pd) | RV100 > 1.3 ml (%) | 57.1 | 0 | NS | |||
160 Gy (I) | 16.7 | 27.6 | NS | ||||
Jarusevicius et al. [7] | 2012 | 230 | 160 Gy | PV100 (%) | 95.5 | 94.9 | 0.21 |
PV150 (%) | 65.3 | 53.2 | < 0.001 | ||||
PD90 (Gy) | 184.7 | 177.9 | 0.002 | ||||
UD30 (Gy) | 218.6 | 197.4 | 0.001 | ||||
RV100 (cm3) | 0.6 | 0.3 | < 0.001 | ||||
HI (%) | 31.8 | 44.0 | < 0.001 | ||||
Ishiyama et al. [8] | 2014 | 140 | 145 Gy | PV100 (%) | 95.7 | 96.6 | NS |
PV150 (%) | 62.1 | 60.4 | NS | ||||
PD90 (Gy) | 170.7 | 174.4 | NS | ||||
UD30 (Gy) | 206.8 | 203.2 | NS | ||||
RV100 (cm3) | 0.51 | 0.47 | NS | ||||
Katayama et al. [10] | 2016 | 64 | 144 Gy | PV100 (%) | 97 | 98.2 | 0.29 |
PV150 (%) | 68.8 | 69.2 | 0.88 | ||||
PD90 (Gy) | 178.1 | 180.7 | 0.29 | ||||
UD90 (Gy) | 154.6 | 165.4 | 0.056 | ||||
RV100 (cm3) | 1.00 | 0.97 | 0.78 | ||||
Hirose et al. [11] | 2017 | 24 | 160 Gy | PD90 (Gy) | 190.3 | 190.1 | 0.98 |
HI (%) | 33.6 | 41.3 | 0.19 | ||||
UD30 (Gy) | 248.9 | 219.9 | 0.16 | ||||
RV100 (cm3) | 0.14 | 0.23 | 0.12 | ||||
38 | 110 Gy + EBRT | PV150 (%) | 80.2 | 62.8 | 0.001 | ||
PD90 (Gy) | 148.3 | 134.5 | 0.004 | ||||
HI (%) | 18.7 | 35.7 | < 0.001 | ||||
UD30 (Gy) | 195.1 | 165.2 | < 0.003 | ||||
RV100 (cm3) | 0.29 | 0.31 | 0.31 | ||||
Inada et al. [12] | 2017 | 74 | 144 Gy or 110 Gy | PV100 (%) | 95.2 | 96.9 | 0.02 |
PV150 (%) | 64.5 | 57.1 | 0.005 | ||||
PD90 (%) | 115.5 | 119.8 | 0.10 | ||||
UD10 (%) | 145.5 | 141.2 | 0.23 | ||||
RD2cc (%) | 64.1 | 61.0 | 0.23 | ||||
Kaneda et al. [13] | 2018 | 76 | 110 Gy + EBRT | PV100 (%) | 98.7 | 98.0 | 0.06 |
PV150 (%) | 66.7 | 51.7 | < 0.001 | ||||
PD90 (%) | 127.2 | 118.8 | < 0.01 | ||||
RV100 (cm3) | 0.61 | 0.44 | 0.03 |
[i] V100 – the percentage of the volume receiving 100% of the prescribed dose, V150 – the percentage of the volume receiving 150% of the prescribed dose, D90 – the minimum dose received by 90% of the volume, D30 – the minimum dose received by 30% of the volume, D10 – the minimum dose received by 10% of the volume, D2cc – the minimum dose received by 2cc of the volume, HI – homogeneity index, LS – loose seed, IBCL – intraoperatively built custom-linked
In the sector analysis, although there were no significantly lower sectors in D90 at 24 hours in the IBCL group, D90 of PIQ at 24 hours in the LS group was significantly lower compared with the other sectors. However, there was no significant difference between the two groups in D90 at 1 month. This may be because LS can change position according to edema of the prostate after seed implantation, and return to the intended position after resolution of the edema; whereas with IBCL seeds, the seeds are less able to move even if edema is present. In their comparison between IBCL seeds and LS, Katayama et al. performed sector analysis and reported improved dose distribution in the anterior base in the IBCL group [10]. Although our method of segmentation is different to theirs, there was no significant difference in the present study between the two groups in terms of D90 and V100 of AIQ at 24 hours or at 1 month.
The prevalence of GU adverse events at 3 months after implantation was significantly lower in the IBCL group than in the LS group. Furthermore, in the comparison using a cut-off value of UD150, the incidence of GU adverse events was significantly lower in patients with lower UD150. These results suggest that high urethral dose is associated with the occurrence of GU adverse events and that fewer GU adverse events occur in the IBCL group because the dose to the urethra was lower in this group. In their studies regarding GU adverse events after brachytherapy, Ishiyama et al. and Katayama et al. found no significant difference in the prevalence of adverse events between the two groups [8,10]. These previous studies evaluated only the presence or absence of adverse events, whereas the present study examined the frequency of adverse events according to the length of follow-up period, which we consider to be a more detailed analysis and the first to show a statistical advantage of IBCL seeds over LS in terms of GU adverse effects. Even though the optimal prescription dose in LDR brachytherapy is controversial, numerous studies have reported a correlation of PD90 and PV100 with prognosis [26,27,28,29,30]. As mentioned above, the present study found no significant difference in PD90 and PV100 between the IBCL and LS groups. Thus, these results suggest that IBCL seeds can reduce urethral dose without lowering PD90 and PV100. Moreover, the use of IBCL seeds may enable the prescription dose to increase without increasing GU adverse events and reduce biochemical recurrence of prostate cancer. These results suggest that IBCL seeds are especially recommended for small prostates. When the prostate is small, there is a limited space within the prostate, in which seeds can be inserted, and reducing the urethral dose is often difficult. Other situations where IBCL seeds may be recommended include combination therapy of brachytherapy and external beam radiation therapy, which also requires reduction of urethral dose.
This study had several limitations. First, it was limited by its retrospective design and single-center setting, a relatively short follow-up period, and a relatively low number of patients. The median follow-up time of 4.2 years in the LS group is considered short for assessing the prognosis of localized prostate cancer. However, no biological recurrence was observed during this follow-up period, and the therapeutic results of both groups may be considered acceptable. Second, CT images were used for dose evaluation. It is well-known that the seeds cause artifacts on CT that make it difficult to contour the margin of the prostate, and that contouring of the prostate varies greatly between practitioners [31,32,33]. To eliminate these effects, we used CT-MR fusion images for contouring. Third, the brachytherapy methods vary greatly among facilities, such as prescription doses, dose limitations for organ at risk, seed insertion techniques, and evaluation periods. Therefore, attention should be given when applying the results of this study to other facilities.
Conclusions
In this retrospective single institutional study, as compared with the LS group, the IBCL group showed a decrease in the SD of the dosimetric parameters, which were statistically significant with respect to change in the variables between 24 hours and 1 month (F-test). The use of IBCL seeds significantly decreased PV150, UV150, and UD30, and significantly improved HI without lowering PD90 and PD100. The prevalence of GU adverse events at 3 months was significantly lower in the IBCL group than in the LS group.