Introduction
Graves’ orbitopathy (GO), also known as thyroid associated orbitopathy (TAO), is the most common extrathyroid manifestation [1]. The main clinical manifestations are exophthalmos, exposure keratitis, and compressive optic nerve. In severe cases, optic neuropathy or corneal detachment occurs. The influence of genetic and environmental factors often leads to autoimmune diseases, and GO incidence is increasing year by year [2]. Currently, the specific pathogenesis of GO has not been fully elucidated [3]. Adipogenesis and lipid accumulation in adipocytes increased the intraorbital tissue volume and led to increased intraorbital pressure, exophthalmos and impaired vision [4, 5]. Therefore, adipocyte differentiation plays an important role in the pathogenesis of GO.
A series of microRNAs (miRNAs) directly or indirectly participate in innate and adaptive immune responses, which may play a key regulatory role in the occurrence and development of autoimmune diseases [6]. MiR-182, a member of the miR-183/96/182 cluster, was first found in the eyes of mice [7, 8]. MiR-182 was highly expressed in mouse retina, and was considered to be an essential miRNA for the genesis and development of sensory organs [9]. Dong et al. confirmed that miR-182 was a negative regulator of adipogenesis [10]. However, the role of miR-182 in preorbital adipose tissue in GO is unknown. E74-like factor 3 (ELF3), an important member of the E-twenty-six (ETS) transcription factor family, plays a role in many pathophysiological processes, such as cancer and immune system disorders [11, 12]. Zhu et al. reported that ELF3 was abnormally down-regulated in GO patients [13]. This suggests that ELF3 may be involved in the pathogenesis of GO. In this study, we found that ELF3 could bind to the promoter of miR-182 through bioinformatics prediction, suggesting that ELF3 stimulated miR-182 to regulate adipogenic differentiation in GO.
The thyrotropin receptor (TSHR) is a G protein coupled receptor found in thyroid follicular epithelial cells, adipocytes, osteocytes and cardiomyocytes [14]. It has been reported that TSHR was upregulated along with intraorbital preadipocytes of GO patients differentiated into mature adipocytes [15-17]. The PI3K/AKT pathway is a known TSHR signal transduction pathway in Graves’ disease [18]. Woeller et al. confirmed that TSHR promoted orbital fibroblast proliferation in GO patients through the PI3K/AKT pathway and aggravated GO progression [19]. Bioinformatics prediction revealed that there was an interaction between miR-182 and the 3’-UTR of TSHR mRNA. We speculated that miR-182 might participate in the development of GO by regulating TSHR expression.
In conclusion, this paper aimed to confirm that ELF3-mediated miR-182 impeded adipogenic differentiation by regulating TSHR to inhibit the PI3K/AKT pathway, thus alleviating GO. This provided a theoretical basis for its clinical treatment.
Material and methods
Source of clinical specimens
The preorbital adipose tissues of GO patients (30 cases) were obtained from severe GO orbital decompression, and the preorbital adipose tissues of the control group (30 cases) were obtained from patients without a history of hyperthyroidism and other immune inflammation. After the tissue specimens were removed during the operation, the clinical samples were frozen with liquid nitrogen. This study was approved by the ethics committee of Xiangya Hospital, Central South University Xiangya Hospital, Central South University and the GO patients signed the informed consent form.
Culture and differentiation of 3T3-L1 preadipocytes
3T3-L1 cells (ATCC, Shanghai, China) were cultured in complete medium (Dulbecco’s Modified Eagle Medium [DMEM] + 10% fetal bovine serum [FBS] + 100 U/ml penicillin and streptomycin, Gibco, NY, USA) in a 37°C incubator (5% CO2, 95% air). The medium was changed every 2 days. Cells were induced to differentiate 2 days after entering the logarithmic growth phase. The culture medium was replaced with differential medium (DM) with 0.5 mmol/l 3-isobutyl-1-methylxanthine (IBMX, Merck, NJ, USA), 1 mmol/l dexamethasone (Dex, Merck) and 5 mg/ml insulin (Merck). After 3T3-L1 preadipocytes were induced to differentiate for 2 days, the differentiation medium was replaced with a maintenance medium containing 10% FBS, 5 mg/ml insulin and DMEM, maintained for 2 days, and then replaced with a complete DMEM medium containing only 10% FBS. The medium was changed every 2 days, and the follow-up experiment was carried out on the 9th day.
Cell transfection
MiR-182 mimics, miR-182 inhibitor, pcDNA3.1-ELF3 (oe-ELF3) vector, pcDNA3.1-TSHR (oe-TSHR) plasmid and the corresponding negative control (NC mimics, NC inhibitor and oe-NC) were all purchased from Genepharma (Shanghai, China). The above plasmids were transfected into cells for 48 h according to the instructions of Lipofectamine 3000 transfection reagent (Invitrogen, NY, USA).
Western blot
RIPA lysate was added to the cells to obtain total protein, and protein concentration was calculated by a BCA protein quantitative kit (Beyotime, Shanghai, China). Appropriate protein dosage was electrophoresed by 10% sodium dodecyl sulfate-polyacrylamide gel (SDS-PAGE) electrophoresis and transferred onto PVDF membranes. Then, the membranes were blocked with 5% skim milk at 25°C for 2 h, and the primary antibody diluent of the corresponding protein was incubated at 4°C overnight. Thereafter, the corresponding secondary antibody was incubated at room temperature for 2 h. Afterwards the bands were incubated with ECL luminescence solution (Thermo Fisher Scientific, USA) and placed in a chemiluminescence imaging system to expose for visualization. The relative expression of each protein was deconstructed using ImageJ software (National Institutes of Health). Antibody information is as follows: PI3K (ab154598, 1 : 2000, Abcam, UK), p-PI3K (ab278545, 1 : 1000), AKT (ab8805, 1 : 500, Abcam, UK), p-AKT (ab38449, 1 : 1000, Abcam, UK), GAPDH (ab181602, 1 : 10,000, Abcam, UK).
Quantitative real-time PCR
Total RNA of 3T3-L1 cells and preorbital adipose tissue were obtained according to the standard procedures in the instructions of TRIzol reagent (Invitrogen). RNA concentration was determined by UV spectrophotometer. Subsequently, the first cDNA strand was synthesized by reverse transcription according to the instructions of the reverse transcription kit (Biosystems, Shanghai, China). Real-time PCR was performed using the PCR SYBR (TaKaRa, Tokyo, Japan) Green method. The relative expression level of gene was evaluated using the 2-∆∆Ct method. GAPDH and U6 were used as an internal control. The primer information is as follows:
miR-182: (F) 5´-GCCGAGTTTGGCAATGGTAGA ACTC-3´,
(R) 5´-GTCGTATCCAGTGCAGGGTCCGAGGTATTCGCACTGGATACGACCGG TGT-3´;
TSHR: (F) 5´-ACTTCGGTCCCTGAAAACGC-3´,
(R) 5´-TGTGCCTGGTGGAAATCAAAT-3´;
ELF3: (F) 5´-TGGCACTGAAGACTTGGTGT-3´,
(R) 5´-CTCTGCAAGGTGGAGTACAGC-3´;
Adiponectin: (F) 5´-GGAACTTGTGCAGGTTGGAT-3´,
(R) 5´-CCTTCAGCTCCTGTCATTCC-3´;
Leptin: (F) 5´-TCACACACGCAGTCGGTATC-3´,
(R) 5´-CACATTTTGGGAAGGCAGGC-3´;
PPAR-´: (F) 5´-AAGAGCTGACCCAATGGTTG-3´,
(R) 5´-ACCCTTGCATCCTTCACAAG-3´;
AP2: (F) 5´-GTCACCATCCGGTCAGAGAG-3´,
(R) 5´-TCATCGACTTTCCATCCCACTTC-3´;
U6: (F) 5´-CTCGCTTCGGCAGCACA-3´,
(R) 5´-AACGCTTCACGAATTTGCGT-3´;
GAPDH: (F) 5´-AGCCCAAGATGCCCTTCAGT-3´,
(R) 5´-CCGTGTTCCTACCCCCAATG-3´.
ELISA assay
Before and after induction of differentiation, 3T3-L1 cells were washed twice with ice saline, 200 µl of normal saline was added to each well, lysed ultrasonically for 1 min, and the supernatant was collected by centrifugation. Triglyceride (TG) content was detected according to the operating instructions of the triglyceride detection kit (Abcam, Cambridge, UK).
Oil Red O staining
The Oil Red dye was dissolved in isopropanol, filtered and placed at 4°C. The cells before and after differentiation were washed twice with 0.01 mol/l phosphate buffered saline (PBS), fixed with 3.7% formaldehyde for 2 min, then washed with water, stained with Oil Red O dye (Solarbio, Beijing, China) for 1 h, and washed until the background was transparent. After Oil Red staining and hematoxylin re-staining, the lipid droplets in the cytoplasm were bright red, and the nucleus was blue. The cell differentiation process was photographed and recorded.
Dual-luciferase reporter gene assay
TargetScan (http://www.targetscan.org/vert_72/) predicted the binding sites of miR-182 with TSHR. The targeting relationship was then confirmed with dual- luciferase reporter gene assay. The 3’-UTR cDNA sequences (including the binding sites to miR-182) were cloned into the pGL3 luciferase reporter gene (Genepharma, Shanghai, China). MiR-182 mimics or NC and TSHR-WT/MUT mimics were co-transfected into cells for 48 h. Luciferase activity was verified following the luciferase report assay (Promega, WI, USA) procedure.
The binding sites of ELF3 to miR-182 promoters were speculated using JASPAR (https://jaspar.genereg.net/). Oe-ELF3 or oe-NC plasmids, reporter gene plasmids and internal reference plasmids were transfected into cells for 48 h. Luciferase activity was verified according to the luciferase report assay (Promega) procedure.
RNA binding protein immunoprecipitation assay
RNA binding protein immunoprecipitation (RIP) was performed using a protein immunoprecipitation kit (Geneseed, Guangzhou, China). Cells were collected and lysed on ice for 10 min. After centrifugation, 100 µl of supernatant was taken, then 900 µl of NET-2 buffer was added to the suspension, and anti-Ago2 antibody or homologous matched control antibody normal mouse immunoglobulin G-bound magnetic beads (Millipore, Massachusetts, USA) were added at 4°C and incubated overnight. After centrifugation, the supernatant was taken and washed with NET-2 to obtain RIP samples. RNA was extracted after protease K digestion (Gibco) and detected by qRT-PCR.
Chromatin immunoprecipitation assay
Formaldehyde was added to 3T3-L1 cell culture medium to cross-link proteins and DNA. Chromatin immunoprecipitation (ChIP) antibody was added and incubated at 4°C overnight to obtain DNA/protein precipitation. DNA was isolated and purified for qPCR. The primer sequences were synthesized by Sangon Biotech (Shanghai, China).
Statistical analysis
Data were analyzed using SPSS22.0 Software (IBM SPSS Statistics, IBM Corp., Armonk, NY, USA). Experimental results were expressed as mean ± standard deviation (all experiments were repeated 3 times). The comparison between the two groups was analyzed by Student’s t-test. Additionally, one-way analysis of variance (ANOVA) was used to ascertain the differences among multiple groups. P < 0.05 was considered statistically significant.
Results
The expression of miR-182 increased and TSHR decreased in GO patients and 3T3-L1 adipocytes
We collected preorbital adipose tissue from 30 patients with GO and non-GO to explore the expression of miR-182 and TSHR. We noted that miR-182 expression was downregulated, while TSHR expression in GO patients was upregulated (Fig. 1A, B). Furthermore, in the analysis of the correlation between miR-182 and TSHR by Pearson’s correlation coefficient, the results showed a negative correlation between miR-182 and TSHR (Fig. 1C). Adiponectin and leptin are adipose factors secreted specifically by adipose tissue, and PPAR-γ and AP2 protein are the main markers of adipocyte differentiation [20]. We observed that the expression levels of adiponectin, leptin, PPAR-γ and AP2 were abnormally increased in the tissues of GO patients (Fig. 1D-G). The expression of miR-182 and TSHR in DM-induced 3T3-L1 cells was ascertained by qRT-PCR assay and the results showed that miR-182 was decreased, while TSHR expression was increased in DM-induced 3T3-L1 cells (Fig. 1H, I). Moreover, it was not difficult to observe that the expression levels of adiponectin, leptin, PPAR-γ and AP2 were abnormally increased in DM-induced 3T3-L1 cells (Fig. 1J-M). In addition, the TG content in DM-induced 3T3-L1 cells was increased (Fig. 1N). The lipid droplet formation in DM-induced 3T3-L1 cells was significantly increased (Fig. 1O). Therefore, the above results suggested abnormal expression of miR-182 and TSHR in vivo and in vitro.
Fig. 1
Expression of miR-182 and TSHR in GO patients and 3T3-L1 adipocytes. A, B) Expression of miR-182 and TSHR in preorbital adipose tissue was determined by qPCR assay. C) Pearson’s correlation coefficient was determined to evaluate the correlation between miR-182 and TSHR. D-G) Adipocytokine expression in preorbital adipose tissue was determined by qPCR assay. The descriptive statistics were presented as mean ± SD. *p < 0.05, **p < 0.01, ***p < 0.001 Expression of miR-182 and TSHR in GO patients and 3T3-L1 adipocytes. H-M) The expression of miR-182, TSHR and adipocytokines in DM-induced 3T3-L1 cells was determined by qPCR assay. N) ELISA assay was manipulated to detect TG content. The descriptive statistics were presented as mean ± SD. *p < 0.05, **p < 0.01, ***p < 0.001 O) Lipid droplet content was ascertained by Oil Red O staining. For each group at least three independent experiments were performed. The descriptive statistics were presented as mean ± SD. *p < 0.05, **p < 0.01, ***p < 0.001
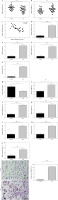
MiR-182 mimics inhibited adipogenic differentiation in 3T3-L1
In order to explore the effect of miR-182 on adipogenic differentiation, we transfected miR-182 inhibitor or miR-182 mimics into DM-induced 3T3-L1 cells. As shown in Figure 2A, miR-182 expression was significantly inhibited by miR-182 inhibitor, whereas miR-182 mimics promoted miR-182 expression. MiR-182 expression in DM-induced 3T3-L1 cells was decreased (Fig. 2B). MiR-182 inhibitor further downregulated miR-182 expression, whilst miR-182 mimics could upregulate miR-182 expression in DM-induced 3T3-L1 cells (Fig. 2B). Then, we found that the expression levels of adiponectin, leptin, PPAR-γ and AP2 in DM-induced 3T3-L1 cells were significantly increased, and miR-182 inhibitor further aggravated this trend; by contrast, miR-182 mimic transfection reduced the levels of adipocytokines (Fig. 2C-F). The TG content in DM-induced 3T3-L1 cells increased significantly and miR-182 inhibitor enhanced TG release, but miR-182 mimics decreased TG release (Fig. 2G). Also, in the Oil Red O staining assay, miR-182 inhibitor promoted the increase of lipid droplets in DM-induced 3T3-L1 cells, while miR-182 mimics had the opposite effect, which reduced lipid droplet production (Fig. 2H). The above results indicated that miR-182 mimics reduced the adipogenic differentiation of DM-induced 3T3-L1 cells.
Fig. 2
MiR-182 mimics inhibited adipogenic differentiation of 3T3-L1. A-F) Expression of miR-182 and adipocytokines was determined by qPCR assay. The descriptive statistics were presented as mean ± SD. *p < 0.05, **p < 0.01, ***p < 0.001 G) ELISA assay was manipulated to detect TG content. H) Lipid droplet content was ascertained by Oil Red O staining. For each group at least three independent experiments were performed. The descriptive statistics were presented as mean ± SD. *p < 0.05, **p < 0.01, ***p < 0.001
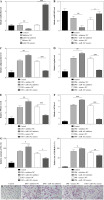
MiR-182 directly regulated TSHR expression
To determine the targeting relationship between miR-182 and TSHR, we found a potential binding site amongst them by TargetScan (Fig. 3A). Dual-luciferase reporter gene assay and RIP assay showed that miR-182 directly targeted to TSHR (Fig. 3B, C). TSHR expression was decreased by miR-182 mimics (Fig. 3D). These results suggested that miR-182 bound to TSHR and negatively regulated its expression.
Fig. 3
MiR-182 directly targeted TSHR. A) TargetScan was used to predict the relationship of miR-182 and TSHR. B, C) Dual-luciferase reporter genes and RIP were used to confirm the targeting relationship. D) TSHR expression was ascertained by qPCR assay. For each group at least three independent experiments were performed. The descriptive statistics were presented as mean ±SD. *p < 0.05, **p < 0.01, ***p < 0.001
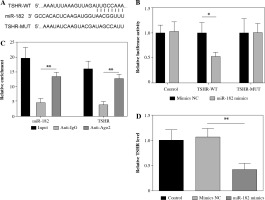
Oe-TSHR reversed the promotion of miR-182 on adipogenic differentiation in 3T3-L1
In order to further explore the role of miR-182/TSHR in DM-induced 3T3-L1 cells, we overexpressed TSHR in DM-induced 3T3-L1 cells by transfecting the oe-TSHR plasmid (Fig. 4A). Then, miR-182 mimics downregulated TSHR expression, while TSHR expression was increased after oe-TSHR in DM-induced 3T3-L1 cells (Fig. 4B). Furthermore, miR-182 mimics significantly inhibited the expression of adiponectin, leptin, PPAR-γ and AP2 in DM-induced 3T3-L1 cells, but oe-TSHR reversed the effect of miR-182 mimics (Fig. 4C-F). Moreover, miR-182 mimics down-regulated the TG release in DM-induced 3T3-L1 cells, whilst oe-TSHR increased TG content and attenuated the effect of miR-182 mimics (Fig. 4G). In addition, we found that the quantity of lipid droplets decreased in DM-induced 3T3-L1 cells with miR-182 mimics, while lipid droplet formation increased when oe-TSHR and miR-182 mimics co-transfected into DM-induced 3T3-L1 cells (Fig. 4H). These results indicated that oe-TSHR attenuated the inhibitory effect of miR-182 mimics on adipogenic differentiation in 3T3-L1.
Fig. 4
Oe-TSHR reversed the function of miR-182 in adipogenic differentiation. A-F) The expression of TSHR and adipocytokines was determined by qPCR assay. The descriptive statistics were presented as mean ± SD. *p < 0.05, **p < 0.01, ***p < 0.001 G) ELISA assay was manipulated to detect TG content. H) Lipid droplets content was ascertained by Oil Red O staining. For each group at least three independent experiments were performed. The descriptive statistics were presented as mean ± SD. *p < 0.05, **p < 0.01, ***p < 0.001
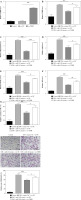
ELF3 transcriptionally promoted miR-182 expression
ELF3 expression was significantly down-regulated in GO patients and DM-induced 3T3-L1 cells (Fig. 5A, B). To determine the targeting relationship between miR-182 and ELF3, we predicted that ELF3 bound to multiple promoters of miR-182 using the JASPAR database (Fig. 5C). ChIP assay and dual-luciferase reporter assay revealed a targeted binding relationship between miR-182 and ELF3 (Fig. 5D, E). The above results suggested that ELF3 bound to the promoter of miR-182 and increased its expression.
Fig. 5
ELF3 transcriptionally promoted miR-182 expression. A, B) ELF3 expression was ascertained by qPCR assay. C) JASPAR was manipulated to predict the relationship of miR-182 promoters and ELF3. D, E) Dualluciferase reporter assay and ChIP were used to confirm the targeting relationship. For each group at least three independent experiments were performed. The descriptive statistics were presented as mean ± SD. *p < 0.05, **p < 0.01, ***p < 0.001
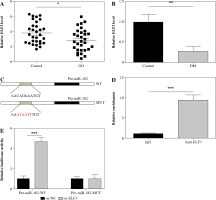
ELF3 stimulated miR-182 involved in the adipogenic differentiation in 3T3-L1 cells
ELF3 expression was increased in 3T3-L1 cells with oe-ELF3 (Fig. 6A). Oe-ELF3 up-regulated miR-182 level and down-regulated TSHR expression (Fig. 6B, C). Then, oe-ELF3 inhibited the expression of adiponectin, leptin, PPAR-γ and AP2 in DM-induced 3T3-L1 cells, but miR-182 inhibitor reversed the effect of oe-ELF3 (Fig. 6D-G). We found that the expression of p-PI3K and p-AKT in DM-induced 3T3-L1 cells was up-regulated, and oe-ELF3 inhibited PI3K/AKT signaling pathway activation, while miR-182 inhibitor reversed the effect of oe-ELF3 (Fig. 6H). Furthermore, oe-ELF3 inhibited the TG release in DM- induced 3T3-L1 cells, but miR-182 inhibitor eliminated the impact (Fig. 6I). in addition, oe-ELF3 decreased the quantity of lipid droplets in DM-induced 3T3-L1 cells, while miR-182 inhibitor alleviated the function of oe-ELF3 (Fig. 6J). These results implied that miR-182 inhibitor attenuated the inhibitory effect of oe-ELF3 on adipogenic differentiation in 3T3-L1 cells.
Fig. 6
MiR-182 inhibitor reversed the inhibitory effect of oe-ELF3 on adipogenic differentiation. A-G) The expression of ELF3, miR-182, TSHR and adipocytokines was determined by qPCR assay. The descriptive statistics were presented as mean ± SD. *p < 0.05, **p < 0.01, ***p < 0.001 H) PI3K/AKT signaling pathway in DM-induced 3T3-L1 cells was ascertained by WB. I) ELISA assay was manipulated to detect TG content. J) Lipid droplet content was ascertained by Oil Red O staining. For each group at least three independent experiments were performed. The descriptive statistics were presented as mean ± SD. *p < 0.05, **p < 0.01, ***p < 0.001
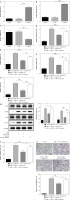
Discussion
The increase of adipose tissue in GO patients can not only directly lead to increased intraocular pressure and exophthalmos, but also, as an endocrine organ, adipose tissue itself can secrete a variety of adipocytokines, growth factors and protein molecules to participate in the occurrence and development of GO [21, 22]. Kumar et al. found that functional representative factors secreted by adipocytes, leptin, adiponectin and PPAR-γ were highly expressed in orbital tissues of GO patients compared with non-GO patients, indicating that adipocytes proliferated abnormally in this process [23]. We found abnormally increased expression of adiponectin, leptin, PPAR-γ and AP2 in preorbital adipose tissue of GO patients and DM-induced 3T3-L1 cells. Furthermore, the contents of TG and lipid droplets increased in DM-induced 3T3-L1 cells and ELF3-stimulated miR-182 could suppress adipogenesis in 3T3-L1 cells by DM induction by regulating TSHR expression.
ELF3 mediated leptin to induce inflammation in articular chondrocytes via the PI3K/AKT pathway [24]. Zhu et al. found that ELF3 expression was abnormally decreased in extraocular muscles of GO patients [8]. Likewise, our study found that ELF3 expression was significantly down-regulated in preorbital adipose tissue of GO patients and DM-induced 3T3-L1 cells. Additionally, oe-ELF3 suppressed the expression of adiponectin, leptin, PPAR-γ and AP2, and inactivated the PI3K/AKT signal pathway in DM-induced 3T3-L1 cells.
MiRNAs are important regulators of immune pathways, and some miRNAs have been found to be dysfunctional in a variety of autoimmune diseases [25], including GO [26]. Recent research showed that miR-27 expression in preorbital adipose tissue of GO patients was significantly down-regulated [27]. Jang et al. confirmed that miR-146a regulated inflammatory protein expression and cell function in orbital fibroblasts and participated in the pathogenesis of GO [28]. MiR-182 was downregulated during preadipocyte differentiation in 3T3-L1, and involved in the regulation of adipocyte differentiation [29]. Jeon et al. found that the lipid synthesis regulator SREBP-2 activated the transcriptional activity of miR-182 promoter to promote its expression [30]. We found that miR-182 expression was abnormally down-regulated in orbital preadipose tissue of GO patients and DM-induced 3T3-L1 cells. MiR-182 mimics decreased expression of adipocytokines (adiponectin, leptin, PPAR-γ and AP2), TG content and lipid droplets, to inhibit the adipogenic differentiation of DM-induced 3T3-L1 cells. Kong et al. reported that ELF3 activation could be directly targeted with miR-1224-5p in pancreatic cancer cells [31]. Interestingly, we similarly found that ELF3 targeted miR-182 promoter and positively regulated miR-182 expression. MiR-182 inhibitor promoted the adipogenic differentiation of DM-induced 3T3-L1 cells and oe-ELF3 reversed the inhibitory effect of miR-182 inhibitor on adipogenic differentiation.
Douglas et al. reported that fibroblasts expressed TSHR and other related autoantigens differentiate into adipocytes, accumulate in the orbital tissue of GO and participate in the pathogenesis of GO [32]. Kumar et al. demonstrated that low concentration of thyrotropin receptor antibody (TRAb) promoted the differentiation of orbital preadipocytes into adipocytes through the PI3K/AKT signaling pathway, playing a central role in adipocyte differentiation [33]. Moreover, TSH or TRAb combined with TSHR activated the cAMP-PI3K-AKT signaling pathway to promote orbital adipose differentiation [34]. In the present study, TSHR expression was abnormally increased in orbital preadipose tissue of GO patients and DM-induced 3T3-L1 cells. TSHR is a target gene of miR-182. miR-182 inhibited adipocytokine expression and reduced the contents of TG and lipid droplets by inhibiting TSHR expression, thereby alleviating the adipogenic differentiation of DM-induced 3T3-L1 cells.
To sum up, we confirmed for the first time that ELF3 transcriptionally mediated miR-182 negatively regulated TSHR to inhibit the PI3K/AKT signal pathway, thereby ameliorating GO progression. This paper might provide a potential theoretical basis for the clinical treatment of GO.