Purpose
High-dose-rate (HDR) or pulsed-dose-rate (PDR) brachytherapy (BT) have demonstrated an essential role in the curative management of many pathologies, including cervical cancer. Excellent control rates of locally advanced cervical cancer (LACC) have been reported with external beam radiotherapy (EBRT) and concurrent chemotherapy, followed by BT [1, 2].
Technological evolution of EBRT and development of new techniques, such as intensity-modulated radiotherapy (IMRT), volumetric modulated arc therapy (VMAT), or stereotactic body radiotherapy (SBRT) have opened a new range of possible treatments for cervical cancer [3, 4]. However, it has been reported that BT produces high-dose distributions in the target, with higher dose gradients delivered and better sparing of critical organs than EBRT [5, 6]. The Society of Gynecologic Oncology (SGO), the Groupe Européen de Curiethérapie-European Society of Therapeutic Radiation and Oncology (GEC-ESTRO), and the American Brachytherapy Society (ABS) in their recent recommendations state that the avoidance of BT for cervical cancer has been related to negative consequences affecting survival, and SBRT should not be a substitute for BT in patients undergoing primary curative intent radiation therapy for cervical cancer [5, 7].
Previously, with 2D BT treatment planning, protocols for cervix BT were based on dose points, such as points A or ICRU (International Commission on Radiation Units and Measurements) points [8-10]. Following introduction of computerized axial tomography (CT), the access to specific anatomy of patients turn out to be possible, and with dose-volume histograms (DVH) information, it became available for target volumes and organs at risk (OARs). However, protocols and tolerances were still based on dose points. In 2005-2006, GEC-ESTRO published recommendations for cervix cancer BT, in which T2-weighted magnetic resonance imaging (MRI) was the recommended image modality due to its’ superior soft tissue contrast, which allowed identification of tumor response during treatment [11, 12]. These guidelines, later collected and supported by the ICRU Report No. 89 [13], introduced and defined a delineation procedure for gross tumor volume (GTV), high-risk clinical target volume (HR-CTV), intermediate-risk clinical target volume (IR-CTV), and OARs, focusing on dose prescriptions and tolerances of 90% and 2 cm3 of these volumes, respectively. Recommendations on dosimetric information, which should be reported included also: reference air-kerma rate (RAKR) of the source, dose to recto-vaginal point and points A, D98 (dose absorbed by 98% of the volume), D90, and D50 of HR-CTV, and D0.1cc, D2cc of the bladder, rectum, sigmoid, and bowel (main OARs).
In 2008, GYN GEC-ESTRO workgroup started EMBRACE (IntErnational Study on MRI-based BRAchytherapy in CErvical Cancer), with a goal of assessing and validating MRI-guided BT. In this first study, dosimetric quantities introduced previously by GEC-ESTRO and later supported by ICRU (e.g., D90, D2cc) were used, and their recommended values were based on historical and clinical experiences of participating institutions [14]. In 2011, a retrospective project (RetroEMBRACE) began with the aim of analyzing previously treated patients to beginning of EMBRACE [15]. With clinical data obtained from both the studies, the objectives of EMBRACE II study were established [16]. In this last protocol [17], the tolerance, objective, and mandatory values of different dosimetric parameters of each of relevant structures were reported, based on clinical evidence [18].
Recently, De Leeuw et al. [19] presented a report concerning a Dutch national quality assurance program for state-of-the-art curative radiotherapy for patients with LACC. This project was useful to show the need for training concerning the adaptation to the new EMBRACE II treatment protocol.
EMBRACE II protocol introduced new tolerance and objective doses for target and OARs volumes. The aim of the present paper was to analyze the evolution of dosimetric outcomes of cervix BT implants in three Spanish institutions, with respect to EMBRACE II publication.
Material and methods
Dosimetric and volumetric data of cervix BT interventions were collected. All data were obtained from three Spanish hospitals, with which the authors are affiliated. A total of 392 patients were analyzed, including 260 from Institution 1, 72 from Institution 2, and 56 from Institution 3. All patients followed a similar treatment scheme: EBRT followed by 4 MRI-based BT fractions applied in two applicator insertions, 7 Gy per fraction, prescribed according to a GEC-ESTRO biological spread sheet as a compromise between D90 to HR-CTV and IR-CTV, and D2cc to OARs. EBRT component scheme varied according to a patient and Institution, and included 45 Gy in 25 fractions, 50.4 Gy in 28 fractions, 50 Gy in 25 fractions, 52.2 Gy in 29 fractions, 46 Gy in 23 fractions, 48.74 in 24 fractions, and 44.8 Gy in 28 fractions. Most common scheme was 45 Gy delivered in 25 fractions.
This study comprised patients from 2009 until 2019, depending on the data available at each Institution. For each patient, total dose and number of EBRT fractions, D90 of HR-CTV, and D2cc of OARs of BT were obtained. In addition, HR-CTV volume (an average of two BT applications) and number of needles used were also recorded.
All three institutions used Elekta BT devices, such as Oncentra TPS (Elekta, Veenendaal, The Netherlands), Utrecht interstitial CT/MR applicator, and HDR-BT afterloader (one Institution had a MicroSelectron v.4, and the other two used Flexitron 1.0). Utrecht applicator combines intracavitary and interstitial gynecological BT. 4 and 6 mm diameter intra-uterine tubes, with 15 and 30 degrees curvatures were combined with 15, 20, and 25 mm ovoids, and up to five interstitial plastic needles in each ovoid, with three located in the outer side and two in the inner part, closer to the tandem [20]. Several patients were treated with tandem and ovoids only.
Even though the EMBRACE II protocol was published in 2018, certain institutions had access to the draft versions prior to that date. Not all hospitals could adapt their established protocols at the same time. Institution 1 adapted the EMBRACE II as soon as they obtained access to a draft version at the end of 2016. Institution 2, due to its’ participation in the EMBRACE I clinical trial, could not completely adjust its’ protocol until end of 2017. Institution 3 has still not completely changed the EMBRACE II objectives and constraints. Furthermore, Institution 3 included the sigmoid within delineation of the rectum structure. For this reason, it was assumed that D2cc of the rectum and sigmoid were equal for all patients at this center.
Since the aim of the study was to assess the evolution of dosimetric quality of BT insertions, all dosimetric numbers were compared to the EMBRACE II objective/ tolerance levels for BT component. This protocol was used as a reference since it adds to the state of knowledge regarding local control of tumors and complications in OARs. Therefore, a dose in EQD2 (equivalent total doses in 2 Gy fractions computed using linear-quadratic model, with α/β = 10 Gy for tumor and α/β = 3 Gy for OARs) of EBRT contribution was subtracted from objective/ tolerance values stated at EMBRACE II (Table 1) to obtain objective/tolerance values for BT. It was assumed that both D90 HR-CTV and D2cc of OARs received EBRT prescribed dose.
Table 1
Objective and tolerance prescription limits for HDR cervix treatment planning according to EMBRACE II protocol. EQD2 includes 45 Gy/25 fractions of EBRT. Table taken from the EMBRACE II publication [16]
The equilibrium between dose coverage of HR-CTV and dose of OARs was assessed using index I defined as:
where D90obj and D2cc,obji are the objective doses (in EQD2) for HR-CTV and tolerance levels for different OAR, and D90lim is the upper limit dose for HR-CTV. It should be noted that this objective and tolerance doses refer only to BT component. For example, if EBRT treatment consisted of 45 Gy with 1.8 Gy/fraction (44.25 Gy EQD210 and 43.2 Gy EQD23), D90obj = 90 – 44.25 = 45.75 Gy EQD210, D90lim = 95 – 44.25 = 50.75 Gy EQD210, and D2cc,objbladder = 80 – 43.2 = 36.8 Gy EQD23. According to this definition, the optimal value of index I was 0. There is a critical case, in which D90 is equal to D90lim, which causes the value of index to be independent of OARs doses. However, with a sufficient decimal precision, this should not occur. In all treatments with D90 higher than D90lim, the index had a negative value. Moreover, the proportion with OARs was kept within negative range, and treatments with a higher absolute value had a worse assessment. D90obj has to be understood as a minimum objective value. Since EMBRACE II established a planning aim of D90 between 90 Gy EQD210 and 95 Gy EQD210, higher doses within this interval were considered in the present study. Consequently, index I reached its’ optimal value, when D90 equals to D90lim, and not when corresponds to D90obj. None of the patients presented with D90 of HR-CTV lower than 85 Gy EQD210.
Statistical analysis
Statistical analysis of all the dosimetric results was carried out using RStudio software version 1.2.1335. RStudio is an environment for R, a programming language for statistical computing. For those institutions, which have adapted to the EMBRACE II protocol, assessment of statistically relevant differences between two periods (i.e., pre- and post-EMBRACE II) was performed. Depending on distribution of characteristics, Welch, Wilcoxon, or Yuen statistical tests were applied. A p-value of < 0.05 was considered statistically significant.
Results
Table 2 presents a summary of the analyzed cohort, and a percentage of pure IC and IC/ IT techniques in both periods.
Table 2
Summary of patients’ cohort. Volume of HR-CTV represents mean between two applications. All uncertainties represent k = 1
Pre-EMBRACE II | Post-EMBRACE II | |||||||
---|---|---|---|---|---|---|---|---|
n | HR-CTV volume (cm3) | IC (%) | IC/IT (%) | n | HR-CTV volume (cm3) | IC (%) | IC/IT (%) | |
1 | 121 | 21 ±13 | 3 | 97 | 139 | 17 ±10 | 2 | 98 |
2 | 46 | 28 ±19 | 66 | 34 | 25 | 23 ±17 | 24 | 76 |
3 | 56 | 62 ±14 | 43 | 57 | – | – | – | – |
Figures 1-3 summarize the values and evolution of D90 of HR-CTV, and D2cc of OARs for each Institution, normalized by objective or tolerance value for brachytherapy component of the treatment. This means, for example, for a patient who received 43.2 Gy EQD23 in EBRT, normalization factor of D2cc rectum would be 21.8 Gy EQD23. However, for the same patient, normalization factor of D2cc sigmoid would be 26.8 Gy EQD23. In any case, quantities plotted in Figures 1-3 are equal to one, when HR-CTV receives exactly 90 Gy10 in HR-CTV, 80 Gy3 in the bladder, 65 Gy3 in the rectum, and 70 Gy3 in the sigmoid after the overall treatment. Boxes in these figures represent the 25th and 75th percentiles, horizontal line shows the median, and extremes of vertical lines, with 95% of distribution.
Fig. 1
Values of D90 and D2cc of HR-CTV and OARs of Institution 1 for pre- and post-period of adaptation to EMBRACE II
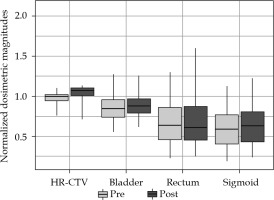
Fig. 2
Values of D90 and D2cc of HR-CTV and OARs of Institution 2 for pre- and post-period of adaptation to EMBRACE II
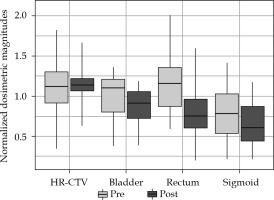
As can be seen in Figure 1, after adapting of EMBRACE II in Institution 1, a conservative approach, increasing the dose to HR-CTV enough to be able to keep OARs almost unchanged, reached a median value of 1.071 for normalized D90. In fact, only D90 was found to present a statistical difference between both periods (p = 0.000003). On the other hand, Institution 2 already approached a high-dose in HR-CTV, and even exceeded in some cases 96 Gy and 95 Gy thresholds established in EMBRACE I and EMBRACE II, respectively. The adaptation to new constraints was translated to a statistically significant reduction of doses to OARs (pbladder = 0.03, prectum = 0.0004, psigmoid = 0.02), and delivery of more concentrated HR-CTV dose between objective and upper threshold, as shown in a smaller box in Figure 2. Figure 4 shows an evolution of D90 HR-CTV and D2cc of the rectum in Institution 2, together with a percentage of interstitial implants with respect to the total number of patients. Since the tolerance limits of OARs were less demanding in the EMBRACE I protocol, distributions of OARs in Figure 3 for Institution 3 were mostly above unity, and distribution of D90 of HR-CTV was below unity.
Fig. 4
Evolution of D90 HR-CTV and D2cc of the rectum in Institution 2, together with the percentage of interstitial implants during the whole analyzed period. Blue and red markers represent treatments during pre- and post- EMBRACE II publication
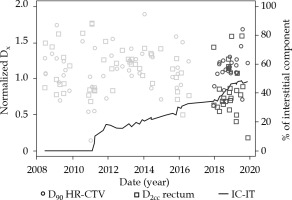
Index I introduced the above evaluates, with an effect of newly established EMBRACE II objectives having worse values for those treatments with a higher absolute value. Figure 5 and Table 3 represent the distribution of index I for the three centers. A tendency towards higher HR-CTV doses in Institution 2 (Figure 2) was interpreted here as a significant percentage of index I distribution being negative. High doses to OARs (with respect to the EMBRACE II constraints) of implants in Institution 3 were expressed by index I with higher values. Index I was significantly different only for Institution 1 (p = 0.0000001) for the two analyzed periods. Even though the median was not statistically different for Institution 2 (p = 0.06), the index I integrated the reduction of variation shown in Figure 2 for both target volume and OARs. Quality of an implant depends on many factors, especially volume of the CTV. The volume of HR-CTV influences the level of conformity and doses to OARs, so that it is possible to achieve without an interstitial component. As can be seen in Figure 6, the equilibrium between D90 of HR-CTV and D2cc of OARs was harder to handle for larger CTV volumes, which was expressed by an increase of index I with volume of the tumor.
Fig. 5
Index I of the three Institutions in the two periods analyzed. Box represents the 25th and 75th percentiles and extremes of vertical lines, including 95% of distribution
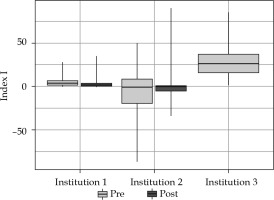
Fig. 6
Dependence of index I with the volume of HR-CTV. Inner graph zooms out the region on volumes lower than 15 cm3
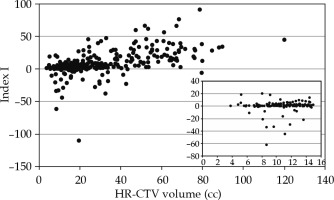
Table 3
Summary of index I distribution for all three centers with two analyzed periods. P-value between analyzed periods is included with significance for values lower than 0.05
Institution | Pre-EMBRACE II | Post-EMBRACE II | |||||
---|---|---|---|---|---|---|---|
Mean | SD | Range | Mean | SD | Range | p-value | |
1 | 6 | 7 | –0.1-46.9 | 4 | 8 | –3.1-65.8 | 0.0000001 |
2 | –5 | 28 | –110.8-59.0 | 2 | 22 | –34.1-90.3 | 0.06 |
3 | 28 | 19 | 1.8-113.9 | – | – | – | – |
Discussion
This study was a retrospective analysis, in which dosimetric information used did not affect patients’ treatments. A total of 392 patients from three Spanish centers, with higher number of cervix BT treatments were analyzed. Most patients were treated from 2012 to 2019. This was a period of numerous developments and published recommendations by international societies. The hypothesis tested was to assess the improvement of both implants and dosimetry planning with time, based on training and experience of both physicians and physicists. The analysis presented in this study showed three different approaches to the above-mentioned adaptation that depended on available resources, adaptation of clinical and optimization protocols, and characteristics of the treated patients among other factors. Institution 1 from the beginning, treated almost all patients with an interstitial component. In this case, the goal was to increase the dose to the tumor while keeping OARs at least as good as in the period of EMBRACE I. Institution 2 evolved from a majority of pure intracavitary implants. The main impact of this change was a statistically significant reduction of doses to OARs depending on the technique used (IC vs. IC/IT; pbladder = 0.02, prectum = 0.003, psigmoid = 0.03). Institution 2 sought a maximum allowed dose to CTV, even overreaching this threshold in some patients. The peculiarity of large tumor volume of patients in Institution 3, and the majority of intracavitary implants (Table 2) led to a subtle evolution and difficult equilibrium between D90 (coverage of tumor) and OARs. The lack of sufficient interstitial components in an implant of above-average HR-CTV volume, could lead to increased tandem loading, resulting in a more cylindrical dose distribution and increased dose to OARs. As can be expected, those implants with interstitial component led to higher coverage and at least equal OARs sparing (pHR-CTV = 0.03). This effect was mainly caused by better management of OARs doses than the interstitial component could provide [20, 21]. In Figure 6, treatments with higher HR-CTV volumes were ranked lower by index I. This was in fact due to the above-mentioned effect and lack of HR-CTV coverage resulting from OARs limitations [22].
Although all three institutions used MRI for contouring purposes, the distribution of HR-CTV volumes was different between centers (Table 2). This difference may be related to different patient referral patterns as well as specific geographical and screening strategies.
The distribution of HR-CTV volumes published varied between studies. The EMBRACE group has published some studies, which included HR-CTV volume statistics. Jastaniyah et al. [23] presented a volumetric analysis of GTVD (gross tumor volume at diagnosis) and HR-CTV defined according to GEC ESTRO recommendations. In this case, the reported mean and standard deviation was 31.6 ±16.1 cm3. Recently, the EMBRACE I group [24] reported that HR-CTV mean volume of 1,416 patients was 28 cm3, with 95% confidence interval (20-40 cm3). Also, in Figure 1, an estimated range of 8-117 cm3 is presented [17].
Other studies with HR-CTV volume statistical data have been also published. Cannon et al. showed [25] their results of 2020 audit, in which mean HR-CTV volume was 19.27 cm3 (range, 9.9-29.33 cm3), and Oud et al. [26] reported HR-CTV average volume of 34 patients as 29.4 ±12.5 cm3 (range, 8.5-92 cm3).
A limitation of our study, aiming at index evaluation, was a lack of contouring uncertainty analysis. In Institutions 2 and 3, a single radiation oncologist performs BT treatments, which confirms the absence of inter-observer variation; on the other hand, in center 1, two radiation oncologists carry out the contouring.
All institutions had a learning and skill curve with regard to the application of interstitial component. Initially, few needles were used, but over time, more and deeper needles were employed not only for enhancing dose conformity to HR-CTV, but also for sparing of OARs. The trend shown in Figure 4 represents this evolution. In Institution 2, significantly lower doses were applied to all OARs when an interstitial component was used (pbladder = 0.02, prectum = 0.003, psigmoid = 0.03, pHR-CTV = 0.3). For Institution 3, the opposite results were found; only the coverage of the target volume was significantly different when needles were included (pbladder = 0.8, prectum = 0.3, psigmoid = 0.3, pHR-CTV = 0.03). In order to understand the difference in OARs doses between Institutions and for each institution, two relevant points must be considered. On the one hand, threshold doses for OARs present significant variations between EMBRACE I and EMBRACE II projects, since the former limits were based on historical experience of only a few institutions, and the latter were supported by clinical evidence. Alternatively, the adaptation of each center’s protocol to new guidelines of the EMBRACE II project was not initiated at the same time. New objectives for HR-CTV coverage and OARs sparing could also mean adapting the optimization process and/ or the necessity to include more interstitial components in learning process to induce changes.
Index I was used as a metric to illustrate the impact of EMBRACE II in dosimetric outcomes of HDR-BT implants. This index endeavors to assess the equilibrium between HR-CTV coverage and OARs sparing, but not to assess individual plans. However, some limitations must be outlined. There was a critical point in the definition. In those cases, in which D90 equals to D90lim, the index equals to 0, independently of OARs doses. This situation was always avoided using decimal precision of the treatment planning system (TPS) and Excel sheets applied for radiobiological calculations. This critical point would make the index non-applicable for institutions or specific patients, where treatment was re-normalized in order to equal D90 to D90lim. It is interesting to analyze individually both terms, i.e., ICTV and IOAR, as compensation between these two components could occur. However, such compensation was uncommon, since only sub-optimal implants (rare anatomy, lack of interstitial component, etc.) led to low doses and poor coverage of HR-CTV, high OARs doses, or high doses to both, target volume and OARs.
Conclusions
Embrace II and ICRU 89 introduced a new paradigm on BT planning towards higher doses to target volumes and OARs better sparing. In order to achieve these new objectives, an increase of interstitial component is needed. However, not all centers had the training or protocol flexibility to adapt to these new guidelines. A total of 392 patients from three Spanish institutions, with a majority of cervix brachytherapy treatments were analyzed. Depending on the local protocol before EMBRACE II, the adaptation was achieved by increasing the interstitial component and the physician and physicist training, which resulted in a significant increase of HR-CTV doses or a reduction of OARs doses. The introduced index I has been able to quantify the evolution of the equilibrium between CTV coverage and OARs sparing, according to EMBRACE II objectives and constraints.