Purpose
Post-mastectomy radiation therapy (PMRT) significantly reduces loco-regional recurrence (LRR) rates and improves overall survival in patients with high-risk breast cancer [1, 2]. The dose regimen for PMRT is usually 50 Gy to the chest wall (CW), followed by an additional 10 Gy to the surgical chest wall scar, especially for patients with close or positive mastectomy margins and large (i.e., ≥ 5 cm) tumors [3]. Radiation therapy in these cases is delivered by several modalities, including high-dose-rate (HDR) brachytherapy and external beam radiotherapy (EBRT). Among EBRT techniques, orthovoltage radiotherapy with beam energies in the range of 100-250 kV, or electrons or high energy photons can be used [4]. With the above-mentioned modalities, the most commonly applied techniques are electrons and surface mould HDR brachytherapy. Usually, (en)-facing electrons are used to deliver the boost dose. However, electron treatment planning and preparation are involved, as electron cut-outs and dose featherings require both the time and effort.
The treatment option is usually based on institutional resources and expertise, which results in local control, cosmesis, and toxicity compliance of the treatment. The most common practice for achieving this is tangential fields by 3D conformal radiotherapy, intensity-modulated radiotherapy (IMRT), or volumetric modulated arc therapy (VMAT), followed by (en)-face electron boost to the scar region. Post-operative scar boost radiation for the reconstructed breast presents many planning challenges due to the shape, size, and curvature of the scar [5]. The advantages of brachytherapy over electrons are a rapid dose fall-off outside the target volume and short treatment duration [6]. There is an extreme lack of studies investigating the role of HDR surface mould brachytherapy scar boost following PMRT, in the setting of close or positive margins [7]. Our experience over many trials in designing the standard mould for scar boost irradiation and air gaps leads to inhomogeneity in dose. An attempt was made to use commercially available flaps for the treatment of chest wall boost. In contrast, boost treatment during adjunct radiotherapy, following breast conservation surgery is documented [8]; however, not much literature is available about effective chest wall boosts during PMRT.
A clinical trial is being conducted in our institute assessing local control and morbidity when treating post-operative chest wall in breast cancer using a combination of external beam radiotherapy and HDR surface mould brachytherapy with a catheter flap. This study proved an effective method of efficacy in dose coverage to tumor volume and sparing the underlying critical organs. In skin HDR brachytherapy, sources are located outside, in contact with the skin surface, or placed within a certain distance from the skin. Most treatment planning systems use the American Association of Physicist in Medicine (AAPM) Task Group (TG) 43 formalism, which is based on single-source dose superposition within an infinite water medium, without accounting for the true geometry, in which conditions for scattered radiation are altered by the presence of air [9].
Acuros® BV is a grid-based Boltzmann solver (GBBS), which directly resolves linear Boltzmann transport equation. It deterministically solves, what Monte Carlo codes solve stochastically. Acuros® BV in BrachyVision version 10.0 reported dose through medium but absorbed dose to water. Absorbed dose to the material was added from version 13.0 onwards. The purpose of this study was to evaluate the dosimetric advantage of using Acuros® BV dose calculation algorithm with 192Ir source for HDR brachytherapy in breast scar boost treatment using catheter flap over TG-43 dose calculations.
Material and methods
Patients’ selection
Patients required a PMRT boost to their chest wall who reported to have close or positive surgical margins, and were free of cardiac and pulmonary co-morbidities were included in this study. Total of 25 patients who met these inclusion criteria were involved in the study. Left-sided breast (n = 11) and right-sided breast (n = 14) patients were planned for external beam radiotherapy with scar boost irradiation using HDR surface mould brachytherapy as a sandwich.
Inclusion and exclusion criteria
Inclusion criteria were as follows: locally advanced carcinoma breast patients (IIIA, IIIB, and IIIC stages), histopathology of infiltrating ductal carcinoma, age < 60 years, ECOG performance score 0 and 1, no chest wall irradiation in the past, no previous history of any cardiac disease and pulmonary dysfunction.
Exclusion criteria were as follows: age > 60 years, ECOG performance score 2 and 3, history of previous radiation to the chest wall, and previous history of any cardiac disease and pulmonary dysfunction.
Catheter flap
Catheter flap® is commercially available (Varian Medical Systems, Palo Alto, CA, USA) device, which measures 200 mm × 290 mm × 10 mm, made of silicon with 20 treatment channels. The mould probe measures 1.8 mm diameter × 320 mm, with made of stainless steel and PA (polyamide-nylon) mandarin. Each channel can be identified with the help of channel marker clips. If required, the catheter flap can be cut to the necessary size, and is used for treating superficial cancers (scar boost irradiation), and the function of the flap is to create a space between the skin and source. The catheters are placed in the middle of the axis flap and spaced 5 mm apart. The probes ensure that the source is placed in a planned position with high precision. Before the catheter flap placement onto the patient skin, biocompatible sterile pads can be placed on patients’ treated area to avoid direct contact with the skin. This sterile pad is less than 1.0 mm, has a minor dosimetric impact, and can be accounted for a bolus to avoid contamination and cross-infection.
Pre-treatment planning
In this study, the patient’s treatment position was maintained in EBRT and surface mould HDR brachytherapy, and documented. Thermoplastic masks were used to immobilize the patient who was placed in a supine position on a carbon fibre breast board, with an ipsilateral arm abducted above the head. This position would help image registration and reproducibility, as a catheter flap for surface mould brachytherapy for scar boost was placed on the chest wall under thermoplastic cast, as shown in Figure 1. Separate planning computed tomography (CT) for EBRT and surface mould brachytherapy was performed before the start of EBRT. Computed tomography simulation using Siemens Somatom Spirit equipment, with 3 mm slice thickness and 1 mm reconstructed image for HDR brachytherapy was performed for every patient.
Target delineation and treatment regime
The contour for all the patients in this study was delineated based on the Radiation Therapy Oncology Group (RTOG) criteria. For HDR surface mould brachytherapy using catheter flap, clinical target volume (CTV) was drawn using radio-opaque (1 mm lead wire) markers placed on the scar, with 3 cm in lateral extension and 3 cm in craniocaudal direction of the scar. Since lead wire can cause attenuation and account for dose difference in Acuros® BV calculation, the Hounsfield units (HU) value for the lead wire was assigned to air equivalent, and were removed during the treatment. A 5 mm thick uniform structure lying beneath the skin, excluding 5 mm from the skin surface, was delineated with boundaries limited to wire markers. A 5 mm thick skin structure was created over the CTV-HDR_evl to restrict the dose constraints during optimization. A dose of 50 Gy in 25 fx (2 Gy/fx) to the chest wall (PMRT) and for supraclavicular lymph node (SCLN) with axillary level of three nodes, and 7.5 Gy/3 fx (2.5 Gy/fx) was delivered by HDR surface mould brachytherapy to the delineated scar volume. The first fraction of HDR brachytherapy was given after seven fractions of EBRT, the second fraction after 14 fractions of EBRT, and the third fraction of HDR surface mould was applied after 21 fractions of EBRT. During the brachytherapy schedule, no EBRT treatment was applied.
Treatment planning algorithms and optimization
All the patients were contoured, planned, and evaluated by a single evaluator to minimize interpersonal variations. All plans were optimized and calculated using Varian BrachyVision (version 13.0), which has both standard TG-43 formalism, and Acuros® BV (version 1.4.0) developed by Transpire, Inc., Gig Harbor, Washington, USA, which was integrated into Eclipse treatment planning system (Varian Medical Systems Inc., Palo Alto CA, USA). Planned treatments were executed in GammaMedPlus® iX (Varian Medical Systems Inc., Palo Alto CA, USA), with a maximum activity of 370 GBq 192Ir stepping radioactive source to deliver the prescribed dose with decay correction. HDR brachytherapy plans were firstly performed using TG-43 formalism and inverse planning adaptive volume optimization. This was achieved with specific objectives for target volume and normal tissue sparing, followed by GBBS algorithm-based calculation (Acuros® BV). During planning, surface mould brachytherapy with catheter flap calculation resolution was set to 1 mm as a standard to provide the best output. Similarly, the matrix grid was identically ensured in both the calculation methods. Both EBRT and HDR surface mould brachytherapy plans were evaluated at the same time in order to arrive at a conclusion on organ dose-limiting and to evaluate skin dose.
Plan evaluation and analysis
All the planning was optimized using an iterative method to obtain desired results with TG-43 formalism. If not achieved, isodose re-shaper tools were used at a particular location to improve the dose coverage. Once the plan was generated, the Acuros® BV algorithm was used without altering the dwell position used in the TG-43 calculation. However, the GBBS algorithm does take into account mass density information for computing dose. Dose distribution was compared between two algorithm plans and analyzed qualitatively and quantitatively with dose-volume histograms (DVHs).
Based on cumulative DVH and differential DVH, the following parameters for target and OARs were obtained:
Dmin, Dmax, Dmean, D2%, D98%, D90%, V100%, V150% for CTV-HDR_evl;
Dmax doses for contralateral breast, contralateral lung, ipsilateral lung, ribs, and skin;
Dmean doses for contralateral breast, heart, contralateral lung, ipsilateral lung, ribs, and skin;
D2% doses for heart and ribs;
V5% and V10% for contralateral breast;
V150% and V200% for skin (volume receiving 150% and 200% of dose in skin structure, which were 5 mm above CTV-HDR_evl).
Calculations were also performed for volumetric homogeneity parameters, including:
coverage index (CI): target volume ref/target volume, where target volume ref is the volume of the target that receives the reference dose;
dose homogeneity index (DHI): 1 – V1.5 ref/target volume ref, where V1.5 ref is the target volume that receives a dose of 1.5 times the reference dose;
overdose volume index (OI): V2.0 ref/target volume ref, where V2.0 ref is the target volume that receives a dose of 2.0 times the reference dose;
dose non-uniformity ratio (DNR): V1.5 ref/target volume ref.
The statistical analysis of mean, standard deviation, 95% CI of mean, and quartile deviation were applied, and plots were performed using IBM SPSS 21 software. Pair t parametric tests were performed comparing two groups of data.
Results
The CTV-HDR_evl volume ranged from 48 cubic centimeters (cc) to 186.4 cc. The number of channels required for the treatment of different volumes of CTV ranged from 10 to 20. Figure 2 shows dose distribution for post-mastectomy scar boost irradiation comparing both TG-43 and Acuros® BV plans. The dose-comparison and its corresponding significance for different parameters in CTV are shown in Table 1. The mean dose for D98% of CTV-HDR_evl for Acuros® BV was 88.19% (SD = 6.6) compared to 96.25% (SD = 7.4) for TG-43. The D2% for CTV showed 138.5% (SD = 12.4) for TG-43 vs. 128.0% (SD = 11.8) with Acuros® BV. The mean D98% CTV-HDR_evl coverage decreased by 10.45% (p = 0.000) and near-maximum dose reduced by 7.56% (p = 0.000) for Acuros® BV plan as compared to TG-43-based calculation. The mean Dmax dose to CTV-HDR_evl and the mean Dmean dose were lesser by 5.85% (p = 0.000) and 9.76% (p = 0.000), respectively, as compared to TG-43. The dose homogeneity index and dose non-uniformity ratio indicated statistically significant results. However, overdose-volume index presented findings that were close to statistical significance.
Fig. 2
Dose-volume histogram comparing TG-43 and Acuros® BV-based planning algorithm for clinical target volume (CTV) and organs at risk (OARs) in chest wall scar boost irradiation using catheter flap
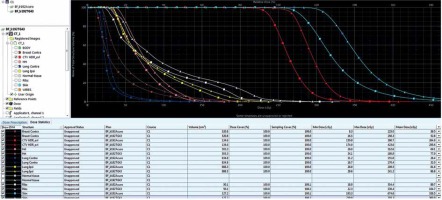
Table 1
Comparison of various parameters of CTV-HDR_evl between TG-43 and Acuros® BV algorithm with 192Ir source
In the contralateral breast, the mean V5% showed a decrease in the dose by 33.76%, and the mean V10% indicated a reduction of 39.35% (p = 0.000) with respect to TG-43-based calculation. The mean V5% and V10% showed very significant differences between the two algorithms, but the maximum dose demonstrated close to statistically significant results (p = 0.04). The heart D2% (cGy) showed a significant outcome, with a decrease in the dose by 5.73%, whereas Dmedian (cGy) presented a very significant difference, with a reduced dose by 9.49% vs. TG-43. The mean dose to the heart with Acuros® BV plan was 81.6 cGy compared to 91.6 cGy with TG-43. In the contralateral lung, Dmean, Dmax, and V5% showed very significant differences compared to Acuros® BV-based dose computation. In this study, the ribs of the diseased side were included for comparison, of which D2% indicated substantial results. The near maximum dose to the ipsilateral ribs with Acuros® BV plan was 198.36 cGy (SD = 51.94) compared to 227.82 cGy (SD = 55.68). A 5 mm thick skin contour was delineated over the CTV-HDR_evl, and the dose difference between the two algorithms showed statistically very significant results (p = 0.000) for V150% and V200%.
In descriptive statistics, the box and whisker plot explained the minimum value, first quartile, median, third quartile, and maximum value, distribution, and skewness of the data. In Figure 3, two algorithms were compared to show respective medians, interquartile ranges, dispersion, overall spread, and signs of skewness, with potential outliers for max doses, mean doses, and near-max doses of OARs. Since the skin dose was significant, V150% and V200% were also compared between TG-43 and Acuros® BV-based calculation. It was evident that all OARs, Dmean, and Dmax values were relatively lower in doses compared to TG-43 calculation. Similarly, Dmin doses also followed the same pattern of results, and a relative reduction in dose was seen with Acuros® BV-based planning. The mean dose plot demonstrated positive skew for the ribs and negative skew for the ipsilateral lungs. The D2% of all OARs indicated, the heart was lower in the dose compared to the ipsilateral ribs with TG-43. When Acuros® BV was used, the dose reduction was observed due to tissue inhomogeneity, which predicted more accurate dose computation. The D2% for the ipsilateral lungs was negatively skewed. The V150% and V200% of the skin indicated positively skewed data for Acuros® BV.
Fig. 3
The box and whisker plot. A) Comparison of max doses of organs at risk (OARs) with TG-43 vs. Acuros® BV algorithm- based planning in post-mastectomy scar boost irradiation. B) Comparison of mean doses of OARs with TG-43 vs. Acuros ® BV algorithm. C) Comparison of D2% of heart, ribs, and ipsilateral lung between TG-43 vs. Acuros® BV algorithm. D) V150% and V200% volume of skin were compared between TG-43 and Acuros® BV algorithm
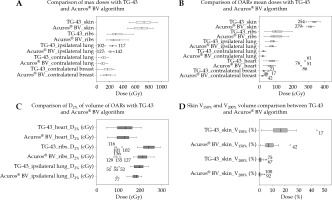
Discussion
In this study, a comparative analysis was carried out to investigate the significance of heterogeneity algorithm in HDR surface mould brachytherapy for chest wall scar boost irradiation using traditional AAPM TG-43 formalism. The vast majority of patients experience isolated chest wall failure and later develop distant metastasis within five years of loco-regional recurrence, with 25% chance of a recurrence after mastectomy for T1-2 N0 disease [10]. The catheter flap application to boost the chest wall scar provides a more conformal and homogeneous dose coverage for the scar volume compared to the electron field. Because the applicator curves with the chest wall can provide a homogeneous dose to the skin surface and 0.5 cm depth, it also avoids the need to use two or more matching electron fields [11]. If HDR surface mould brachytherapy is used for surgical scar boost as post-mastectomy radiotherapy, then accounting for an accurate dose prediction is possible with Acuros® BV algorithm. The catheter flap for scar boost irradiation is enclosed by immobilization cast, which ensures reproducibility over fractions and minimizes air gaps between skin and catheter flap. Sandwiching HDR surface mould brachytherapy using catheter flap between EBRT is a relatively safe and practical approach for post-mastectomy patients, with reasonable local control.
Treatment planning system (TPS) used in the TG-43 formalism-based on sources within an infinite water medium, without accounting for the scatter defect due to surrounding air [9]. The uncertainties related to the HDR brachytherapy dose calculation model (TG-43) should be acknowledged if HDR brachytherapy is used. The use of Acuros® BV dose calculation model would reduce this uncertainty. The presence of additional air gaps due to the moulds positional variation (from planned) would lead to underdosing the target volume, as the distance from the source positions is increased compared to the planned positions. In our simulations using Acuros® BV dose calculations, the addition of a 4- or 10-mm air gap between the mould and the skin decreased the dose by brachytherapy [12]. These two valid deficiencies and drawbacks were addressed in this study; firstly, by incorporating a thermoplastic mask over the surface mould to eliminate the air gap between the mould and the skin surface, helping in reproducibility within acceptable uncertainties with adequate coverage of prescription dose to the target volume, as shown in Figure 4. Secondly, taking heterogeneity algorithm Acuros® BV for dose computation accounts for actual scatter condition and the surrounding environment. Both Acuros® BV and advanced collapsed cone engine (Elekta, Stockholm, Sweden) have been shown to agree within 2% with Monte Carlo method calculations for single-source models [13], and specifically near the skin for breast brachytherapy patients’ models [14]. The dose difference at the prescription depth (1 cm below the central catheter) increased with the growing treatment area. The dose overestimation of the TG-43 model decreased when a bolus was added above the treatment catheters [12]. This may not be a significant issue when heterogeneity algorithms are accepted in clinical use [15]. Comparison of GBBS and TG-43 formalism on breast interstitial metal catheters shows that the estimated dose to CTV was only marginally different from the two systems. There is a significant difference in estimated doses, ranging from 4% to 53% in the mean value of all parameters analyzed [16]. However, this uncertainty in HDR brachytherapy dose calculation is much more considerable than that observed in external beam dose calculations in the current clinical practice. Presently, the American Brachytherapy Society (ABS) working group [17] recommends using bolus as a backscatter material for skin HDR brachytherapy treatments.
Fig. 4
A) Delineated organs at risk and clinical target volume with catheter reconstruction for scar boost irradiation. B) Clinical target volume enclosing the prescribed dose. C) The comparison of isodose distribution between TG-43 and Acuros® BV-based calculation in transverse, frontal, and sagittal planes
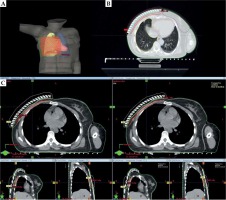
In this study, CTV-HDR_evl received the prescribed dose as the mean value for V100% of 70% (SD = 15.6) and their minimum dose was 67% (SD = 4.01) with Acuros® BV-based planning; these doses were 25.6% and 10.9%, respectively, lesser compared with TG-43-based planning. The dose coverage to 98% of the CTV-HDR_evl volume (D98%), respectively, with their mean values of 86.19% (SD = 1.3), which were 10.4% reduced than TG-43-based dosimetry. These differences were noted without altering the dwell positions and achieved reduction due to actual scatter conditions. The mean dose (SD) to the heart was 0.91 Gy (12.4), which was 63.3% of the prescribed amount (PD) with TG-43-based calculation, and with Acuros® BV-based calculation it was 0.81 Gy (11.23), which was 67% of the prescribed dose (PD). A difference of –3.7% was observed in the Acuros® BV calculation to the prescribed dose. These results are comparable with mean cardiac doses reported by a similar study (Dmean of 0.9 Gy with TG-43 dosimetry) [7]. The dose receiving a 2.0% volume was 129.4 cGy with standard calculation and 122.02 cGy with Acuros® BV-based dosimetry, –5.7% decrease was noted comparing with the current dosimetry technique.
The mean ipsilateral lung dose (MLD) was 76.49 cGy (SD = 3.25, range, 36.6-96.3 cGy) with heterogeneity algorithm, which was –5.7% lesser compared with the TG-43 algorithm. Dmax and D2% values were 214.5 cGy (SD = 6.3, range, 119.9-259.8 cGy) and 176.7 cGy (SD = 5.18, range, 94.41-208.8 cGy), which were about 85.8% and 70.6% of the PD, respectively. These values were –10.9% and –5.8% reduced compared to TG-43 formalism-based calculation. However, combining EBRT and HDR surface mould brachytherapy using catheter flap doses when added, the lung tolerance doses were within the tolerance value of V30Gy < 20% and V20Gy < 30%, respectively. The mean dose to the skin was 311.04 cGy (SD = 3.33, range, 265.7-339.8 cGy), and the volume receiving 150% and 200% doses were received by 8.39% (SD = 0.99, range 0.96-23.89%) with TG-43 algorithm and 0.76% (SD = 0.20, range 0.01-4.22%) with Acuros® BV algorithm. These doses were –7.4%, –50%, and –39% lesser compared to TG-43-based calculations, and the values were significant. Higher doses to the skin were in expected lines that alter the scar boost dose from conventional 1000 cGy to 750 cGy.
It is shown that the HDR surface mould brachytherapy with EBRT was a feasible alternative to EBRT alone in the treatment of chest wall irradiation. Our results demonstrate that OARs doses were lower with Acuros® BV, which accurately considers the tissue inhomogeneities and scatter conditions. We have previously demonstrated that the dose difference between TG-43 and Acuros® BV calculations rises with increasing loading area for HDR brachytherapy surface mould treatments, resulting in a lower actual delivered dose than that seen with TG-43 dose calculation model [13]. The expected benefit of Acuros® BV in dosimetry planning is in the amount of reduction it would achieve (through the individualization of patient’s dosimetry) in a variance of response of clinical trial population [18].
Conclusions
Surface mould brachytherapy for scar boost irradiation combined with EBRT provides many advantages, particularly an alternative to electron beam therapy, and results are comparable in terms of local control rates, with a marginal increase in acute skin reactions. It also helps in reducing treatment time and treatment reproducibility. Large, irregular, and curved areas can be treated. The data comparing TG-43 formalism and Acuros® BV algorithms calculation in scar boost irradiation of breast are limited. This study shows how alternate planning algorithms account for the mass density of the medium, scatter condition, and actual dose prediction in a medium. The study also presented a reduced dose to associated organs and structures, which provides to report the correct dose delivered.