Purpose
Local recurrences in rectal cancer have proven difficult to cure, while causing debilitating symptoms [1]. About three decades ago, the widespread introduction of total mesorectal excision (TME) as standard treatment of rectal cancer has drastically decreased the risk of loco-regional recurrence [2]. For more advanced tumors, a neo-adjuvant treatment with short-course radiation or long-course chemoradiation are often used, resulting in an additional decrease in loco-regional recurrence risk [3, 4]. Often, patients who develop a local recurrence have previously been irradiated, limiting possibilities for high-dose re-irradiation due to toxicity to structures, such as blood vessels, rectal wall, sacrum, and sacral nerves. A lower total radiation dose, for example 15 × 2 Gray (Gy) in combination with oral capecitabine, has proven feasible and effective in a number of studies for patients with a previously irradiated local recurrence [5-7]. Nevertheless, local control remains difficult to achieve, and prognosis of patients with a local recurrence remains low. A microscopically or macroscopically involved resection margin is one of the most important predictors of local recurrence [8-10]. The majority of local recurrences occur without the presence of simultaneous distant metastases, suggesting that preventing and/or treating local recurrences has the potential to decrease morbidity. It is therefore clear that the main aim when treating locally advanced tumors as well as local recurrences, is to obtain an R0 resection by maximal downstaging.
Intra-operative radiotherapy (IORT), delivery of irradiation during surgery, has been developed as a tool to provide a high-dose radiation boost to a limited volume of patients, in whom microscopically involved resection margins are likely to occur. The main advantage of this irradiation technique is its’ ability to deliver a high-dose of radiation to an area at high-risk for tumor involvement, by allowing for direct tumor/risk area visualization and excluding, wherever possible, dose-limiting structures; therefore, limiting dose to surrounding healthy tissue [11].
IORT for rectal cancer can be delivered using electron beam radiotherapy, further referred to as intra-operative electron beam radiotherapy (IOERT), high-dose-rate (HDR) brachytherapy (IOBT), or by using low energy X-rays (kV-IORT). The last technique is currently used occasionally, and will therefore not be discussed further in this paper [11]. A recently published study compared outcomes for both methods (IOERT in Catharina Cancer Institute [CCI] in Eindhoven, The Netherlands, and IOBT in Erasmus Medical Center [EMC] in Rotterdam, The Netherlands) in patients with an R1 resection for locally advanced and locally recurrent rectal cancer [12]. Results from this paper showed a significantly better local recurrence-free survival favoring IOBT. Many factors may have contributed to this result, including different patients’ populations, varying irradiated volumes, differences in operating times as well as differing applicator types. These differences were accounted and adjusted for as much as possible. However, IOBT, due to its’ physical principles, also results in a higher surface dose. Residual microscopic disease should theoretically be located mainly on the surface of resection plane, as this is what distinguishes a microscopically radical versus irradical surgery. Therefore, arguably, IORT should achieve a surface dose as high as possible, without increasing the risk of complications.
In this paper, a detailed description of dosimetric aspects was given of both IOERT and IOBT techniques. Furthermore, due to a potential impact of higher surface dose, we described an adaptation of IOERT technique, narrowing the difference in dose delivery for both methods. The steps required to safely implement this change as well as a review of available literature on the subject were presented.
Background
General dose considerations
The IORT dose when given as a boost, varies from 10 to 20 Gy, depending on the status of residual disease. Recently, ESTRO/ACROP have published recommendations regarding radiation doses for primary locally advanced and locally recurrent rectal cancer [13, 14]. In the case of negative resection margins (R0), the recommended dose ranges from 10 to 12.5 Gy. With a microscopically positive margin (R1), the recommended dose is 12.5-15 Gy. For macroscopic residual disease (R2), the recommended dose is 15-20 Gy [13, 14]. However, caution is warranted due to the tolerance of surrounding healthy tissue [11, 15]. For clarity, in this article, we will be focusing on a dose of 10 Gy.
IOERT technique
IOERT, often delivered by a mobile linear accelerator, is currently the most frequently used technique. Set-up is considered easy, and treatment time is short. It has as an added advantage that the depth of radiation dose can be modulated according to the desired tissue depth treated by choosing the appropriate electron energy. These energies can range from 6 to 20 MeV, but 6 and 9 MeV are most commonly used for rectum IORT [16]. Due to low electron energies used, mobile linear accelerators are safe in most existing operating rooms from a radiation protection safety standpoint [15]. In terms of the treatment itself, due to limited mobility of the mobile linear accelerator, the patient and the accelerator must be moved toward each other. Usually, this involves a rotation or tilt of OR table. Incidentally, the patient’s position must be changed. For example, when the prostate capsule is the area at risk, the patient may have to be moved from prone to supine position, in order to allow for adequate radiation of this area at risk [15].
The main drawback of IOERT lies in its’ applicators that have a fixed, limited diameter, often ranging from 4 cm up to sometimes 10 cm, depending on an institute’s unit model and available accessories [16]. Furthermore, the applicators are usually a circular or elliptical in shape, making them less suitable for curved or narrow areas [15]. These curved areas and irregular surfaces may be the cause of air gaps within the area to be irradiated, which can have repercussions for the dose given in IOERT. The presence of air gaps can be at least partially compensated for by increasing the amount of monitor units given during radiation. Figure 1 below shows an illustration of an IOERT applicator that has been brought into the abdominal cavity and against the presacral region.
Fig. 1
Position of an IOERT applicator within the patient. IOERT applicator has been brought into the abdominal cavity and placed against the presacral region at the location of potential microscopic disease
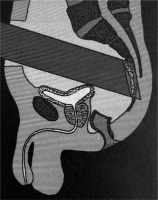
At the Catharina Cancer Institute (CCI) in Eindhoven (NL), IOERT is delivered via a Mobetron 2000 [12] (IntraOp Medical, Sunnycale CA 94085), one of the commercially available mobile linear accelerators. The available energies at CCI are 6, 9, and 12 MeV. For rectum IORT, several circular applicators (from 5 to 7 cm) are available with bevel angles of 30 or 45 degrees. The nominal dose-rate is 10 Gy/min, so maximum radiation time is only a few minutes.
All IOERT data and figures in this article correspond to a 6 MeV beam, a 5 cm diameter, and 45 degree bevel applicator, as it is most commonly used at CCI for rectal cancer, unless specified otherwise. Similar data for other combinations of applicator and energy are available in the Supplementary Material.
IOBT technique
Owning to the fact that a dedicated linear accelerator in the operating room is expensive, and keeping in mind the disadvantage of rigid IOERT applicator shapes and sizes with respect to the complex anatomical surfaces, the use of IOBT is an alternative technique. In IOBT, the radiation is delivered using a HDR remote afterloader. Radio-active source, usually composed of 192Ir, is attached to a thin cable. Source’s positions and amount of time in each position (termed ‘dwell time’) are controlled by a computer, resulting in desired dose distribution [17]. A shielded room is required for radiation protection of the staff in surrounding rooms. Transport of the patient during surgery is not necessary, but radiation times including planning are significantly longer than for IOERT, averaging between 15-60 minutes [17].
The applicator design must be rigid enough to secure the dwell position stability and the integrity of catheters, through which the source cable will travel, but must also be flexible enough to be able to correspond to the anatomical risk area. To obtain the required flexibility, most applicators are composed of silicone. There are several different forms of applicators. Each one warrants a dose prescription that is specific to the form of the applicator. One frequently used model is Harrison-Anderson-Mick (HAM) applicator ((Mick-Radio-Nuclear Instruments Inc., Mount Vernon, NY, USA) that uses embedded catheters. Source-guide tubes are at a distance of 5 mm from the surface of applicator. Therefore its’ dose is often prescribed at 5 mm from the surface of applicator [17]. Another frequently used applicator is Freiburg flap applicator (Nucletron, Veenendaal, The Netherlands), which uses a mesh of connected plastic spheres, each with a diameter of 1 cm that form a flexible applicator. The effective distance from the source to the applicator surface and to the prescription depth are the same as for the HAM applicator.
In the Erasmus Medical Center (EMC) in Rotterdam (NL), the applicator used is a flexible intra-operative template (FIT) consisting of a 5 mm thick silicone flap, through which a maximum of 18 parallel catheters can be placed at 1 cm spacing from one another, and with a maximal length of 22 cm (Figure 2). The FIT applicator can easily be cut to the required size and shape, and is pressed against the at-risk surface using gauze. During surgery, clips are placed to delineate target area [18]. Reconstruction of brachytherapy catheters and creation of a radiation treatment plan occur in treatment planning system. The dose is delivered using a Flexitron HDR afterloader in a shielded OR.
Fig. 2
Image of flexible intra-operative template (FIT) showing 5 mm thickness and spacing of catheters at 1 cm within silicone flap. Additionally, an example of a cut corner is shown [18]
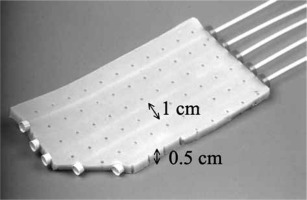
All IOBT data in this article correspond to a FIT with 6 catheters, which was optimized to deliver 10 Gy at 1 cm distance from the FIT surface over a target area of 5 cm × 7 cm, unless otherwise specified. This is comparable to the target area for a 5 cm, 45 degree bevel IOERT applicator.
Description of dose specification methods
As mentioned above, dose geometries are different for the two methods of IORT. For IOBT, the source is brought very close (approximately 2.5 mm) to the tissue at risk, and the source emitted radiation in all directions. For IOERT, the ‘source’ was approximately 50 cm from the tissue within the mobile accelerator, and radiation is delivered using an electron beam in a single direction. Since radiation with electrons is characterized by a build-up area, the surface dose is lower than the dose deeper in the tissue, illustrated in Figure 3.
Fig. 3
Dose depths curves and profiles for IOERT and IOBT. A) Absolute depth dose curves for IOERT (dashed-line) and IOBT (solid line) at clinical axis, being the axis perpendicular to the tissue surface where the central axis of the beam intersects with the tissue surface. B, C) The relative dose profiles at a plane through the center of applicators for IOERT (B) and IOBT (C). For IOBT (C), the catheter points (red dots) and FIT (blue bar) are indicated
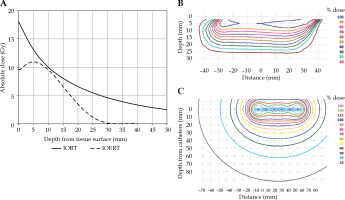
For IOBT, the maximum dose is at the surface of the tissue, after which the dose quickly drops, and then follows a low gradient dose fall over a substantial depth. For IOERT, the dose is about 90% at the surface, and a maximum is reached at 5 mm when using 6 MeV and a 5 cm 45 degree bevel applicator, or deeper for higher energies, shown in Figure 3A. After this, the dose steadily drops to almost 0% within a few centimeters.
The prescribed dose is 10 Gy at CCI and EMC. However, the dose is specified in different ways due to physical properties of each method. For IOBT, a dose of 10 Gy is prescribed at 1 cm from the FIT surface [18]. For IOERT, 10 Gy is specified at the 90% isodose [19]. Normalization point is at the maximum dose at the clinical axis.
In IOERT, dose ‘hot spots’ typically are not higher than 110-120%, while in IOBT, hot spots of 150-200% are always present, though limited in volume. The recommended dose uniformity is 5% within the target volume. For prescription depths of more than 5 mm from the applicator surface, keeping the uniformity at this level as well as the surface dose below 200% often proves challenging [20]. Figure 3 indicates dosimetric differences in current clinical practice.
Goal
Figure 3 illustrates that dose distributions for both methods are very different, even though the prescribed doses are similar.
Recent clinical data [12] suggest that the difference in clinical outcome between IOERT and IOBT may partially be explained by the difference in dose distributions between IOERT and IOBT.
Therefore, in this paper, we proposed a simple adaptation of the IOERT technique and dose prescription, resulting in more comparable dose distributions and potentially, an improvement of clinical results of IOERT in rectal cancer.
Material and methods
Subsequent technique modifications
At CCI, a technique has been developed to increase surface dose for IOERT, with the aim of minimizing difference in dose delivery between IOERT and IOBT. Necessary changes that would be implemented to achieve this result were described below and illustrated in Figure 4.
Fig. 4
Graph showing the current situations for IOERT and IOBT, in absolute dose depth for IOBT (solid line) and IOERT (long-dashed line). Short-dashed line illustrates the IOERT curve after applying a bolus, but before re-scaling. Dotted line shows the IOERT curve with bolus and re-scaling to deliver 10 Gy at 9 mm again
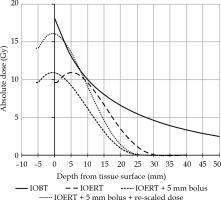
By placing a bolus made of tissue-equivalent material (polymethylmethacrylate, PMMA) at the exit of the applicator, the dose at the designated target area (in this case tissue surface) was increased. This concept is a common practice for other radiation treatments, where an increased skin dose is desired. The build-up that normally occurs within the first few millimeters of tissue, would then occur within the bolus instead, and the dose maximum would move closer to the surface of to-be-irradiated tissue. The ‘IOERT + 5 mm bolus’ curve in Figure 4 illustrates this step. For practical purposes, to be able to do a clean comparison of the techniques, the surface dose was defined as the dose at 1 mm tissue depth.
Consequently, the target tissue was covered by a different part of dose distribution and to preserve/retain the specified dose of 10 Gy at the same tissue depth; for the same combination of diameter, bevel, and energy, the dose would have to be re-scaled (‘IOERT + 5 mm bolus + rescaled dose’ in Figure 4). This was simply done by correspondingly increasing the number of monitor units and therefore the irradiation time. By doing this, the desired effect of increasing the surface dose while maintaining the original dose at relevant prescription depth, thereby reducing the dosimetric differences between IOBT and IOERT, was achieved.
With these changes, the dose at 1 mm from the surface can for example be increased from approximately 9.8 Gy to 15.8 Gy for the 5 cm, 45 degree bevel applicator, using a 6 MeV beam.
Another effect of applying a bolus was that the dose falls back to almost 0% at a shallower depth. The magnitude of this shift equaled the water equivalent thickness of the bolus, and 5 mm PMMA corresponded to 5.7 mm water.
Table 1 gives an overview of the doses at several relevant depths in the tissue for IOBT and IOERT with and without bolus, for the combination of a 5 cm, 45 degree applicator, and a 6 MeV beam.
Table 1
Absolute and relative doses for IOBT and IOERT with the old and new techniques (6 MeV, 45 degree bevel, 5 cm applicator). Relative dose for IOBT was calculated in reference to the dose specification point (10 mm tissue depth). Relative doses for IOERT were reported according ICRU standards, 100% being defined at dose maximum. All depths were specified in target tissue, thus calculated from the outer surface of bolus
As an example, for the 5 cm 45 degree bevel angle 6 MeV beam, a dose of 10 Gy was specified at 9 mm depth in tissue. With the addition of a 5 mm bolus but without re-scaling, the dose at this depth would decrease to approximately 7 Gy (ranging from 90% to 63%). By increasing the number of monitor units by 43%, the dose at 9 mm would be 10 Gy again. The dose at the surface would then increase from ~11 Gy to ~16 Gy.
As a consequence of the standardized method of reporting (ICRU), the specified dose at the 90% isodose, which was a fixed point on PDD, would be 14.7 Gy. This point was now located at the tissue surface and no longer at a relevant depth for prescription. It is therefore important to emphasize that the dose at 9 mm tissue depth was still 10 Gy, as was delivered for the historical patients’ group. Moreover, in addition to the surface dose being higher, the doses beyond 9 mm were lower than in the historical IOERT patients’ group, and still much lower than in the IOBT patients’ group.
It should be noted that IOERT gives an added option of delivering a specified dose of 10 Gy at a greater depth by increasing the beam energy, e.g., in the case of suspected deeper tumor infiltration. At CCI, this happens in about 23% of the cases. On the other hand, depending on patient’s anatomy and radiation equipment limitations, other diameters and bevel angles can be applied. When a 30 degree bevel applicator has to be used, the dose profile is inherently very different from the preferred 45 degree bevel. A 30 degree bevel applicator is used in about 14% of patients.
The main intention for applying the bolus is to increase the dose at the surface of the tissue, but it also allows for the possibility to tailor the dose curve better by simply choosing a different bolus thickness and adjusting the prescription depth. In this way, the delivered dose profile would stronger be based on medical decisions and no longer be dictated by technical limitations.
Table 2 gives an overview of the used combinations between 2018 and 2020. For all these combinations, new applications have been optimized with respect to bolus thickness and prescription depth in order to obtain a similar surface dose and dose curves that were distinguished by the applied energy. A table of relevant parameters using different energies, bevel angles, and bolus thickness can be found in the Supplementary Material.
Follow-up
Patients were followed up according to standard of care every three months with standard work-up. For the first 15 patients treated with the adapted IOERT technique, the radiation oncologist performed intensive monitoring for this new cohort after the surgery to specifically assert potential toxicity due to the increased surface dose, for example plexopathy, bleeding, or other acute effects. In addition, at three, six, and twelve months, patient’s potential toxicity were compared to that of two case-matched patients in the CZE IORT database from the last 5 years to detect an increase in toxicity.
Discussion
Voogt et al. recently observed an improved LRFS for IOBT as opposed to IOERT, and a likely contributing factor for this outcome was the higher surface dose achieved by IOBT [12].
This article illustrates a proposal to increase surface dose for IOERT in patients with primary or recurrent rectal cancer, with the ultimate goal of improving local control in these patients. Increasing the surface dose additionally allows for a smaller difference in dose characteristics for the two methods and enabling easier comparison of the techniques in the future.
The standardized method of reporting (ICRU and ESTRO guidelines) for IORT states that the dose is to be specified at the 90% isodose. In traditional IORT, the energy is selected such that the target tissue is covered by the 90% isodose line. By prescribing to that line, the prescribed dose consequently is also the minimum dose to the target. Inherently to the used techniques, the theoretical dose distribution is as homogeneous as possible within the target tissue, between 90% and 100%, corresponding to 10 Gy and 10.8 Gy, respectively. In reality, though, the actual dose distribution would be influenced by e.g., air gaps and tissue curvature, and therefore be more heterogeneous.
By shifting the dose curve into the bolus, this 90% isodose, which is a physical property, also shifts more superficially. The depth of 90% isodose then no longer represents a relevant depth for dose prescription. It is important to emphasize that while we proposed to increase the surface dose, we would keep the dose at the prescription depth in the patient at the same level, namely 10 Gy.
On the other hand, in order to allow for comparison with past and future techniques as well as with other institutes for research purposes, the absolute dose at the 90% isodose level (e.g., 9 mm for 6MeV) would remain useful and should still be reported. In parallel to prescribing and reporting for stereotactic radiotherapy, we suggest prescribing to the ‘conventional’ tissue depths and in addition, report the minimum and maximum dose within the target tissue as well as the absolute dose at the 90% isodose level along with the respective depths in tissue. The minimum dose to the target tissue would, by definition, be equal to the prescribed dose.
We have mainly focused on the dose profile at the center of the applicators for both IOERT and IOBT. Across the treated area, the dose profile is inherently different for both techniques. This is not altered by adding the bolus. In the paper comparing results of IOERT and IOBT in two Dutch centers, more major complications were seen in patients with LRRC receiving IOBT compared with IOERT (46% and 26%, respectively, p = 0.017) [12]. This outcome may raise concern as to whether the higher amount of complications is related to the higher surface dose given by IOBT. However, we do not expect a significant increase in acute and late toxicity for our adapted IOERT technique. Three important differences between the techniques seem to be that, firstly, for IOBT, much higher hot spots occur along the edges of the FIT and directly under the catheters. Secondly, the dose falls to almost 0% at a much shallower depth for IOERT. Thirdly, irradiated volumes for IOBT are usually larger than for IOERT. A Memorial Sloan Kettering Cancer Center (MSKCC) series described a median treatment area of 30 cm2, but ranging from 4 cm2 to 225 cm2 [21]. In addition, operating times are longer for IOBT due to the treatment planning and other steps occurring during surgery. Despite these observations, a meta-analysis from 2013 showed no significant differences between IOBT and IOERT [22]. We will closely monitor patients for increased toxicity, by implementing extra consultations, during which standardized questionnaires on relevant complications (CTCAE v.5 [23]) will be provided at several times after the treatment. However, due to the relatively small number of patients, the heterogeneity of patients’ group and the expected high complication rate in patients operated for locally recurrent rectal cancer may render determination of the added effect of IORT difficult.
Determination of the cause of toxicity is frequently hampered due to the combination of multivisceral resections, pre-irradiated pelvic anatomical structures, and IORT given, which may all attribute to a certain complication or side-effects. Some side effects, such as peripheral neuropathy and ureteral stenosis, may be more IORT-related than others [21]. In a gynecological IORT setting, in which similar dose levels are used with often corresponding treated areas, two Mayo Clinic series showed that IORT solely contributed to toxicity in 10% and 29% of patients [24, 25]. Similarly, IORT given during rectal surgery has been shown to increase rates of post-operative surgical site infection as well as formation of abscesses in the surgical site [26]. The above-mentioned meta-analysis showed that studies predominantly reported wound-related problems (with incidences up to 46%), gastrointestinal complications, such as fistulae, obstruction of ureter(s), and late neuropathy [22]. Another study also stated that IORT contributed to increased rates of post-operative urological and gastrointestinal complications [27]. One retrospective study found no significant difference in complication rates between a group receiving IOERT and a group without IORT [28]. Unfortunately, there are no prospective randomized trials comparing these two groups of patients. Keeping in mind that many different factors influence the results, the ability to draw conclusions is limited. The above-mentioned MSKCC study shows no association between IORT dose level and incidence of toxicity [21]. One paper described dose escalation within IOBT-treated volume by method of dose-painting, in which a larger volume received a median dose of 15 Gy, and a boost volume received a median dose of 17.5 Gy, prescribed at 0.5 cm from the applicator surface. 19% of patients (in total, n = 3) received a grade 3 complication related to IOBT, being a ureteral stricture, a pelvic abscess and fistula, and a rectovaginal fistula [29]. Despite the fact that it remains difficult to discern IORT-induced toxicity from other causes of toxicity in this complex patients’ group, the data seems to be in line with IORT toxicity data without dose escalation.
Tolerance of healthy tissue has historically been determined by animal (mostly canine) studies. One paper reporting on animal experiments at the Colorado State University has translated these to estimated tolerated doses when using IOERT and EBRT [30-34]. These studies have shown that the maximum tolerated dose of healthy tissue was quite high. For example, the maximum tolerated dose for IOERT was estimated to be 25 Gy single-dose for the ureter (endpoint was radiographic abnormalities) and 15-20 Gy IOERT + 50 Gy EBRT for the lumbar bone (endpoint was bone necrosis) [23-27].
Regarding peripheral nerve tolerance, an analysis was performed by the Mayo Clinic for 56 patients with primary colorectal cancer, who received EBRT, resection, and IOERT. Grade 3 neuropathy was reported in zero patients who received 12.5 Gy or less, 5% of patients who received 15 or 17.5 Gy, and 22% in patients who received 20 Gy or higher in addition to EBRT [35]. Important to note is that it was not specified whether the neuropathy was treatment- or tumor-related. A Spanish group published a paper on long-term normal tissue effects showing that peripheral nerve toxicity was the predominant toxicity attributed to IOERT [36]. The American Brachytherapy Association recommends a single-dose of equal to or less than 12.5 Gy for IOERT for patients with rectal cancer in order to reduce the risk of neuropathy [20].
Surface dose is only one of several differences between dose distributions of the two methods. Other differences leading to a lack of dose are caused by air gaps, tissue curvature, less possibility to correctly place a rigid applicator than a flexible flap, incorrect size of rigid applicator, e.g. due to limited space for positioning (note that in IOERT, the applicator diameter must be 1 cm larger than target), and more. Aside from the difference in dose distributions, the other differences between IOERT and IOBT described in the beginning of this paper remain relevant and need to be considered when formulating any conclusions based on LRFS in the future. One important change is that, with the new IOERT technique, dose-wise compensation of a potential air gap between the applicator and target area is not necessary, as the bolus is fastened to the applicator and air gap would occur in between the bolus and target area.
A major difficulty faced in IORT treatments is the lack of image-guided dose planning. An improvement might be possible due to new in-room imaging devices, including medPhoton ImagingRing m [37], which are currently being developed and improved.
As far as the authors are aware of, no other papers have compared the outcomes of the two techniques. One paper was found describing outcomes of IOERT and IOBT using HDR brachytherapy, and IOBT using LDR 125I seeds for recurrent colorectal carcinoma in a single-institute [38]. However, the authors emphasize that because the choice for each technique was dependent on different factors, comparison of the techniques was not possible.
Conclusions
To the authors’ knowledge, this is the first paper comparing the dosimetric properties of these two techniques. By adding a bolus, we have succeeded in increasing the surface dose while maintaining the dose at the historically prescribed depth. With these adaptations, the depth dose profile of IOERT approaches that of IOBT superficially, but retains the steep dose fall-off past the target tissue. Having optimized this technique, the IOERT treatment is less dependent on patient’s anatomical factors. As a result, we expect an improvement of LRFS without increasing complication rates, which is a subject of further research.