Introduction
Colorectal cancer is one of the most frequently diagnosed neoplasms globally. Although various new therapeutic strategies have been proposed, it remains the second most common cause of cancer-related death in the world [1]. The overall 5-year survival for colorectal cancer stays quite low as many patients are often diagnosed with an advanced stage, therefore limiting the therapeutic options [2]. Surgery is considered to be the only radical strategy [3]. In addition to surgery, therapeutic strategies include chemotherapy, immunotherapy, and photodynamic therapy (PDT) [4]. However, the median survival rate of advanced colorectal cancer still remains low [4].
In the last decade, immunotherapy has been proven to be a potential option to treat colorectal cancer treatment. However, colorectal cancer maintains immune escape by expressing low immunogenicity and following the tolerogenic cell death pathway [5]. Immunogenic cell death (ICD) refers to a specific type of cancer cell death that is induced by various treatments, including certain chemotherapeutic drugs, oncolytic viruses, physicochemical therapies, PDT, and radiotherapy [6]. During the process of ICD, damage-associated molecular patterns (DAMPs) are released, thereby activating the organism’s immune responses. Specifically, ICD inducers can activate unfolded protein responses during the pre-apoptotic stage, leading to endoplasmic reticulum (ER) stress through activation of the protein kinase R-like endoplasmic reticulum kinase (PERK) and phosphorylation of the translation initiation factor eIF2α. Consequently, global protein translation is hindered. Capitalizing on this phenomenon, ER-resident chaperone proteins, including calreticulin (CRT), are translocated to the outer cell membrane via exocytosis. Once at the cell membranes, CRT interacts with the transmembrane receptor CD91 on immature dendritic cells (DCs) and macrophages, triggering a therapeutic immune response known as the “eat me” signal. Furthermore, phosphatidylserines are released, supplementing the interaction with immature DCs and macrophages. Adenosine triphosphate (ATP) is secreted during cell death, attracting DCs by binding to the P2RX7 receptor, also known as the “find me” signal. Additionally, nuclear high-mobility group box 1 (HMGB1) protein is secreted due to cell membrane damage, enhancing cellular permeabilization. In the extracellular space, HMGB1 binds to toll-like receptor 4 (TLR4), activating the myeloid differentiation primary response gene 88 (MyD88) [7]. Collectively, these events facilitate the recruitment of DCs and macrophages, antigen processing, maturation, and antigen presentation to T cells. Ultimately, the immune system is thereafter activated.
Oxaliplatin plays an irreplaceable role in colorectal cancer treatment. The traditional view holds that oxaliplatin suppresses cancer via a cytotoxic effect, which also limits the application of oxaliplatin due to its toxic side effects. Recent evidence has also proved that oxaliplatin is able to induce ICD [8]. Photodynamic therapy has emerged as a promising approach for the treatment of various cancers [9]. It is a non-invasive therapeutic modality that utilizes the combination of a photosensitizing agent, light of a specific wavelength, and molecular oxygen to selectively destroy cancer cells while minimizing damage to healthy tissues. The application of PDT in cancer treatment has garnered significant attention due to its targeted nature, minimal side effects, and potential for synergistic combination with other treatment modalities [10-12]. Furthermore, PDT has shown potential in combination with other treatment modalities, such as chemotherapy, radiotherapy, and immunotherapy, to create synergistic effects and improve overall treatment outcomes. PDT has been proven to be able to promote anti-tumor immunity via ICD [13]. Immune cells, including mast cells, neutrophils, and monocytes/macrophages, act as key contributors in PDT-derived destruction of cancerous cells. Combination therapy is an efficient strategy to reduce drug side effects. However, the effect of PDT combined with oxaliplatin on colorectal cancer remains elusive.
We herein sought to investigate the potential influence of the combination therapy on the proliferation and apoptosis of colorectal cancer cells. More importantly, we evaluated whether DVDMS-derived PDT could synergize with oxaliplatin to strengthen the anti-tumor immune response. Our findings elucidated a novel and potential strategy for colorectal cancer treatment.
Material and methods
Cell culture and in vitro treatment
The human colorectal cancer cell lines DLD-1 and HCT116 were purchased from the Cell Bank of Shanghai Institute of Biochemistry and Cell Biology, Chinese Academy of Sciences and CT26 was originally obtained from American Type Culture Collection and was preserved and cultured by our laboratory. All cells were cultured in Dulbecco’s modified Eagle medium (DMEM) with 10% fetal bovine serum, 100 U/ml penicillin, and 100 mg/ml streptomycin at 37°C in an atmosphere of 5% CO2. Mycoplasma was detected by the Mycoplasma Real-Time PCR Detection Kit (4460623, Thermo Fisher).
For oxaliplatin (O9512, Sigma-Aldrich) treatment, cells were treated with 5 µM oxaliplatin for 4 hours. For PDT treatment, cells were incubated with the complete medium with 1 µM sinoporphyrin sodium for 4 hours, followed by exposure to the 10 J/cm2 laser. For the combination treatment, cells were incubated with 1 µM sinoporphyrin sodium and 1 µM oxaliplatin for 4 hours, followed by exposure to the 10 J/cm2 laser.
Cell Counting Kit-8 assay
Colorectal cancer cells were seeded at a density of 1 × 103 cells per well in a 96-well-plate. After adhesion, cells were treated with 5 µM oxaliplatin, or the 10 J/cm2 laser, or 1 µM sinoporphyrin sodium, or PDT treatment, or PDT + oxaliplatin. The Cell Counting Kit-8 (CCK-8) assay was performed at 0 hours, 24 hours and 48 hours. For CCK-8 assay, CCK-8 (C0038, Beyotime) was added to each well, followed by incubation at 37°C in an atmosphere of 5% CO2 for an additional 4 hours. Absorbance was measured at a wavelength of 450 nm.
Colony formation assay
Colorectal cancer cells were seeded at a density of 1 × 102 cells per well in a 6-well-plate. After adhesion, cells were treated with 5 µM oxaliplatin, or the 10 J/cm2 laser, or 1 µM sinoporphyrin sodium, or PDT treatment, or PDT + oxaliplatin. Then cells were further incubated at 37°C in an atmosphere of 5% CO2 for another 10 days. Cell colonies formed in each well were stained with crystal violet.
Western blot
After treatment, cells were washed with cold phosphate-buffered saline (PBS) and lysed with radioimmunoprecipitation assay (RIPA) buffer supplemented with Mini EDTA-free Protease inhibitor and phosphatase inhibitors. After denaturation, proteins were fractionated by 10% sodium dodecyl sulfate-polyacrylamide gel electrophoresis (SDS-PAGE) and then transferred to PVDF membranes. After blocking the membranes with 5% bovine serum albumin and sequentially incubating with primary and secondary antibodies, the signal detection was performed with ECM Plus Western Blotting substrate. The density of the bands was measured with ImageJ software.
Annexin V/PI assay
Cells were cultured in a 6-well plate. After treatment, all the cells including the culture medium were collected according to the instructions of the Annexin V/PI cell apoptosis detection kit (Bio-Rad Laboratories). Cells were stained with Annexin V for 5 min in the dark, followed by staining with PI for 2 min. Finally, cell apoptosis was analyzed using flow cytometry.
Enzyme-linked immunosorbent assay
Colorectal cancer cells were seeded in a 6-well-plate. After adhesion, cells were treated with 5 µM oxaliplatin, or 10 J/cm2 laser, or 1 µM sinoporphyrin sodium, or PDT treatment, or PDT + oxaliplatin. Then cells were incubated at 37°C in an atmosphere of 5% CO2 for an additional 4 hours. Conditioned medium was collected and stored at –80°C. The ATP and HMGB1 contents in supernatants were measured with respective enzyme-linked immunosorbent assay (ELISA) kits.
Tumor vaccination assay
BALB/c mice were housed in individually ventilated cages (IVC) under SPF conditions with ambient temperature (25 ±5°C) and 45 ±5% relative humidity with a 12-hour light/dark cycle. The animal experiments were approved by the Animal Ethical and Welfare Committee, The Second Xiangya Hospital, Central South University, P. R. China. Mice were randomly separated into 4 groups. Mice in groups a and c were injected with PBS and mice in groups b and d were injected with CT26 cells (1 × 106 cells in 100 µl PBS) pre-treated with oxaliplatin into the right flank of immunocompetent eight-week-old BALB/c mice (day –7). Subsequently, all mice were re-inoculated with untreated tumor cells into the contralateral flank (day 0). Mice in groups c and d were administered 1 mg/kg DVDMS through the tail vein, followed by irradiation with 60 J after photosensitizer administration (day 5). All mice were monitored until sacrificed.
Statistical analysis
All results in graphs are shown as the mean value ± SEM. For the statistical analyses. Statistical analysis was performed with GraphPad Prism. The results were analyzed using an unpaired t-test for two-group comparisons. One-way analysis of variance (ANOVA) followed by Tukey’s test was used for multiple-group comparison. P-values less than 0.05 were considered significant.
Results
DVDMS-derived photodynamic therapy enhances oxaliplatin-induced apoptosis in colorectal cancer cells
Photodynamic therapy has been reported to be used as an alternative therapy for various malignancies, including ovarian cancer [14], breast cancer [15], and liver cancer [16]. By combining light with non-toxic photosensitizers, PDT induces cytotoxic reactive oxygen species in cancer cells, leading to cell death [17]. Sinoporphyrin sodium (DVDMS), a next-generation photosensitizer derived from Photofrin, exhibits potent anticancer efficacy at low doses [15]. In this study, we investigated the impact of DVDMS-derived PDT and oxaliplatin on the viability of colorectal cancer cells. We conducted a CCK-8 assay to assess cell viability after treatment with DVDMS-derived PDT or oxaliplatin. As expected, treatment with laser or DVDMS alone did not affect cell viability, and both DVDMS-derived PDT and oxaliplatin significantly reduced cell viability (Fig. 1A). Additionally, the combination of PDT with oxaliplatin exhibited the highest anti-tumor efficiency among all groups, as demonstrated by the CCK-8 assay and colony formation assay (Fig. 1A-E). These findings suggest that the combined strategy of DVDMS-derived PDT and oxaliplatin synergistically inhibits the viability of colorectal cancer cells.
Fig. 1
The combination of DVDMS-derived photodynamic therapy (PDT) and oxaliplatin depressed the viability of colorectal cancer cells. DLD-1 and HCT116 cells were treated with oxaliplatin, or laser or DVDMS alone, or PDT, or the combination of PDT and oxaliplatin. A) CCK-8 assay was performed at 0 h, 24 h and 48 h to detect the cell viability. B-E) Colony-forming potential was assessed in colorectal cancer cells. (Data are shown as mean ±SD for three independent experiments. *p < 0.05, **p < 0.01 and ***p < 0.001, ****p < 0.0001)
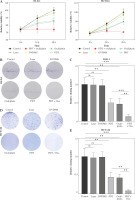
Photodynamic therapy generates molecular oxygen, resulting in the oxidation of biomolecules such as DNA and proteins, ultimately leading to apoptosis [18, 19]. Oxaliplatin has also been reported to induce apoptosis in various tumors [20]. To verify whether DVDMS-derived PDT and oxaliplatin induce apoptosis in colorectal cancer cells, we examined the expression of apoptosis-related proteins. Bax, a key pro-apoptotic protein, plays an essential role in apoptosis [21] and is regulated during programmed cell death [22]. We observed increased expression of Bax after individual treatment with PDT or oxaliplatin. Interestingly, the combination treatment of PDT and oxaliplatin dramatically increased Bax expression (Fig. 2A). In contrast, the expression of Bcl-2, a key anti-apoptotic protein, decreased significantly (Fig. 2A), indicating that the combined treatment enhanced apoptosis. Moreover, flow-cytometric analysis also revealed that the apoptotic rate was significantly increased in the combination treatment group (Fig. 2B). Collectively, our observations suggest that DVDMS-derived PDT combined with oxaliplatin reduces the viability of colorectal cancer cells through apoptosis.
Fig. 2
The combination of DVDMS-derived photodynamic therapy (PDT) and oxaliplatin induced apoptosis in colorectal cancer cells. DLD-1 and HCT116 cells were treated either with oxaliplatin or PDT or the combination of PDT and oxaliplatin. A) Expression of Bax and Bcl-2 was measured by western blot. (Data are shown as mean ±SD for three independent experiments. *p < 0.05, **p < 0.01 and ***p < 0.001, ****p < 0.0001)
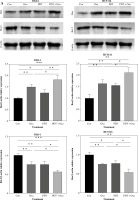
Photodynamic therapy and oxaliplatin combination promotes autophagy in different colorectal cancer cell lines
Autophagy plays a regulatory role in cell homeostasis and metabolism [23] and is crucial for the release of ATP associated with immunogenic cell death [24]. Multiple autophagy-related genes are involved in autophagosome formation, including autophagy-related 5 (Atg5). Atg5 is essential for autophagic flow and facilitates the conjugation of microtubule-associated protein light chain 3 (LC3) to phosphatidylethanolamine, resulting in the formation of LC3-II. LC3-II interacts with various adaptor proteins in the autophagosomal membrane, thereby influencing the autophagic process. To evaluate autophagy in colorectal cancer cell lines, we analyzed the expression of Atg5 protein using western blot in DLD-1 and HCT116 cell lines. Interestingly, treatment with DVDMS-derived PDT or oxaliplatin slightly upregulated the expression of Atg5. Furthermore, the combination treatment resulted in significantly higher levels of Atg5 protein compared to individual administration of PDT or oxaliplatin (Fig. 3). These findings demonstrate that the combination of PDT and oxaliplatin promotes autophagy in different colorectal cancer cell lines.
Fig. 3
DVDMS-derived photodynamic therapy (PDT) amplified the oxaliplatin-induced autophagy. Expression of autophagy- related protein was measured by western blot after treatment in human colorectal cancer cell lines. (Data are shown as mean ±SD for three independent experiments. *p < 0.05, **p < 0.01 and ***p < 0.001, ****p < 0.0001)
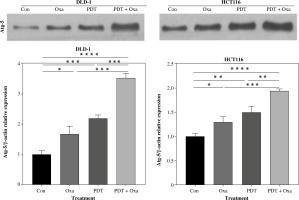
DVDMS-derived photodynamic therapy and oxaliplatin induce the release of ICD markers in human colorectal cancer cells
Immunogenic cell death is characterized by the translocation of calreticulin (CALR), which stimulates antigen transfer to dendritic cells [25]. CALR exposure is closely associated with the phosphorylation of eIF2α. In this study, we found that the expression of p-eIF2α was slightly upregulated after individual treatment with PDT or oxaliplatin (Fig. 4A). However, the combination treatment significantly increased p-eIF2α expression 2.2-fold in DLD-1 cells and 3.2-fold in HCT116 cells (Fig. 4A).
Fig. 4
ICD markers were released in human colorectal cancer cells after treatment with DVDMS-derived photodynamic therapy (PDT) and oxaliplatin. A) Expression of p-eIF2α (Ser51) was measured by western blot after different treatment in human colorectal cancer cell lines. B) Histograms present the alteration of released ATP and HMGB1 in the supernatant of colorectal cancer cell lines in different groups by ELISA assay. (Data are shown as mean ±SD for three independent experiments. *p < 0.05, **p < 0.01 and ***p < 0.001, ****p < 0.0001)
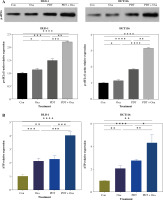
Tumor cells undergoing immunogenic cell death express danger-associated molecular patterns (DAMPs), including surface expression of calreticulin (CRT), secretion of ATP, and release of HMGB1. CRT acts as an “eat me” signal to dendritic cells, triggering phagocytosis [26]. ATP released by tumor cells attracts cytotoxic T lymphocyte (CTL) infiltration [27]. HMGB1 promotes dendritic cell maturation and accelerates antigen presentation to CTLs [28]. We found that the secretion of ATP in the supernatants of different colorectal cancer cell lines was significantly increased after the combination treatment (Fig. 4B). Similarly, the release of HMGB1 was also significantly enhanced after treatment with DVDMS-mediated PDT and oxaliplatin (Fig. 4C). The upregulation of secreted ATP and HMGB1 indicates that PDT can augment the release of oxaliplatin-induced ICD markers. Consistent with previous reports, our results demonstrate that the combination of DVDMS-mediated PDT and oxaliplatin induces immunogenic cell death in vitro.
Fig. 4
Cont. C) Histograms present the alteration of released HMGB1 in different groups. (Data are shown as mean ±SD for three independent experiments. *p < 0.05, **p < 0.01 and ***p < 0.001, ****p < 0.0001)
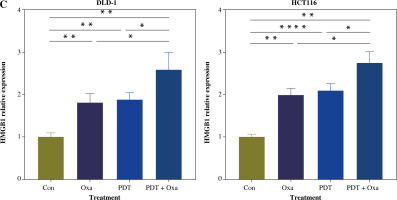
Encouraged by the effects of DVDMS-derived PDT in human colorectal cancer cells, we investigated the role of DVDMS-derived PDT and oxaliplatin in the murine adenocarcinoma cell line CT26. We analyzed the variation in cell viability and observed a significant decrease in absorbance at 450 nm in the combination group, indicating that PDT and oxaliplatin attenuated the viability of CT26 cells. The number and size of foci were also significantly decreased after treatment (Fig. 5A, B). These results indicate that the combination of DVDMS-derived PDT and oxaliplatin decelerates the viability of CT26 cells.
Fig. 5
DVDMS-derived photodynamic therapy (PDT) augmented the oxaliplatin-induced ICD in murine adenocarcinoma cell line CT26. CT26 cells were treated with oxaliplatin, or laser or DVDMS alone, or PDT, or the combination of PDT and oxaliplatin. Cell viability was measured by CCK-8 assay (A) and colony formation assay (B). C) Expression of p-eIF2α (Ser51) was measured by western blot after treatment in CT26 cells
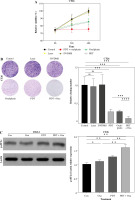
Next, we analyzed the expression of ICD-related markers in CT26 cells. Western blot analysis showed that the combination therapy increased eIF2α phosphorylation, which was 1.44-fold and 1.25-fold more efficient than oxaliplatin and PDT treatment alone, respectively. ELISA analysis of supernatants revealed a 2-fold higher ATP level in the combination group compared to the oxaliplatin or PDT group (Fig. 5D). Similarly, the level of HMGB1 was significantly upregulated in the combination group (Fig. 5E). These findings indicate that DVDMS-derived PDT can enhance oxaliplatin-induced ICD in the murine adenocarcinoma cell line CT26 in vitro.
DVDMS-derived photodynamic therapy and oxaliplatin combination exhibits antitumor vaccination effect in vivo
Indisputable evidence has already shown that ICD markers were released after treatment of DVDMS-derived PDT and oxaliplatin, yet ex vivo experiments cannot replace the vaccination test in vivo. Thus, we introduced BALB/c mice to study the immunogenic potential in the vaccination test. Mice were injected with PBS or CT26 cells (1 × 106 cells in 100 µl of PBS) pre-treated with oxaliplatin into the right flank of immunocompetent eight-week-old BALB/c mice (day –7). Subsequently, all mice were re-inoculated with untreated tumor cells into the contralateral flank (day 0). Mice in groups c and d were administered with PDT (day 5).
We observed that vaccination with the CT26 tumor cells pretreated with oxaliplatin showed moderate tumor growth inhibition efficacy, as did the PDT treatment group. Excitingly, the combination group exhibited the highest antitumor efficiency in all groups, and almost completely eradicated the growth of the primary tumors, indicating that combination-treated CT26 cells stimulated a strong protective immune response in vivo against the subsequent tumor cell challenge. By contrast, mice in the control group showed a negligible tumor rejection effect. Meanwhile, the tumor-free survival was also increased in comparison to the control group (Fig. 6B). At the endpoint, all mice developed tumors in the control group, whereas only one mouse presented a small tumor in the combination group. More interestingly, four mice developed a medium size tumor in both the oxaliplatin group and the PDT group (Fig. 6B, C). These results suggest that the combination of DVDMS-derived PDT and oxaliplatin can induce immunogenic cell death in CT26 cells and subsequently elicit a systemic anti-tumor immune response in immunocompetent BALB/c mice.
Fig. 6
The combination of DVDMS-derived photodynamic therapy (PDT) and oxaliplatin exerts an antitumor vaccination effect in vivo. A) Experimental protocol of the tumor vaccination assay. B) Tumor growth curves of the CT26 primary tumors in BALB/c mice. C) Representative images of the transplantation tumor
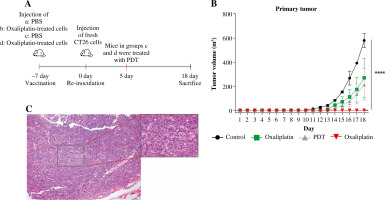
Discussion
The present study aimed to elucidate the role of oxaliplatin and DVDMS-derived PDT in inducing immunogenic cell death in colorectal cancer. Our findings demonstrated that the combined treatment of DVDMS-derived PDT and oxaliplatin resulted in an elevation of phosphorylated eIF2α expression in both DLD-1 and HCT116 cells, leading to a reduction in protein synthesis [29]. In addition, our analysis revealed an increase in the release of damage- associated molecular patterns (DAMPs), including HMGB1, which stimulates dendritic cell maturation, and ATP, which attracts T lymphocytes, in the supernatants of treated cells. Notably, in an anti-tumor vaccination model, vaccination with tumor cells pretreated with the combined treatment exhibited robust inhibition of subsequent tumor cell inoculation in vivo. In summary, our findings suggest that DVDMS-derived PDT can enhance the protective immune response induced by oxaliplatin through the promotion of immunogenic cell death. This highlights the potential of combining DVDMS-derived PDT and oxaliplatin as a novel therapeutic approach for colorectal cancer.
DVDMS, an effective component of Photofrin, has been granted independent intellectual property status in China [30]. Its photochemical activity is 10 times higher than that of Photofrin [31], the first photosensitizer approved by the US Food and Drug Administration (FDA). This makes DVDMS an efficient and safe photosensitizer for cancer PDT [32]. Previous studies have demonstrated that DVDMS-derived PDT can effectively suppress tumor cell proliferation and migration in various malignancies, both in vivo and in vitro [33, 34]. Oxaliplatin, a third-generation platinum drug widely used in colon cancer, primarily kills cancer cells through platinum-DNA adducts [35]. Traditionally, it was believed that chemotherapeutic drugs inducing tumor cell apoptosis were non-immunogenic and did not elicit an inflammatory response. However, recent studies have revealed that certain chemotherapies, including oxaliplatin, can induce immunogenic cell death [36]. A previous study has already demonstrated that PDT can augment the therapeutic efficiency of PtIV nanoparticles in triple-negative breast cancer [37]. In line with previous studies, in our research we also observed that oxaliplatin inhibited the proliferation of human colorectal cancer cells. Interestingly, when combined with DVDMS-derived PDT, the viability of colorectal cancer cells was further reduced, indicating that DVDMS-derived PDT enhanced the inhibitory effect of oxaliplatin on cancer cell viability.
Bax and Bcl-2 are members of the Bcl-2 family, which plays a central role in regulating programmed cell death [38]. Bax is a core regulator of the intrinsic pathway of apoptosis [39]. Activation of Bax leads to permeabilization of the mitochondrial outer membrane, a crucial step in apoptosis [40]. Bcl-2, an anti-apoptotic protein, is also a key regulator of apoptosis [41]. In our study, we observed an increase in Bax-1 expression and a significant decrease in Bcl-2 expression after the combined treatment of PDT and oxaliplatin, indicating enhanced apoptosis resulting from the combination treatment.
To experimentally demonstrate that the combination of DVDMS-derived PDT and oxaliplatin could induce immunogenic cell death in vivo, we performed a vaccination assay using the mouse colon adenocarcinoma cell line CT26. Consistent with the results from human colorectal cancer cell lines, we observed upregulation of predominantly ICD-related biomarkers upon the combination of DVDMS-derived PDT and oxaliplatin. Mice vaccinated with CT26 tumor cells pretreated with this combined strategy exhibited significant suppression of subsequent tumor cell challenges, indicating the successful establishment of an anti-tumor immune response under these conditions.
In summary, our results illustrate the role of DVDMS- derived PDT and oxaliplatin in inducing immunogenic cell death in colorectal cancer. The combination of DVDMS-derived PDT and oxaliplatin contributes to the inhibition of cancer cell viability in vitro. Importantly, DVDMS-derived PDT augments oxaliplatin-induced immunogenic cell death and subsequently elicits a systemic anti-tumor immune response in vivo. Understanding the role of DVDMS-derived PDT and oxaliplatin in colorectal cancer is crucial for facilitating the development of innovative cancer therapies. Overall, these findings provide a potential therapeutic strategy against colorectal cancer.