Purpose
Ruthenium-106 (106Ru) brachytherapy is used to treat choroidal melanomas up to approximately 6 mm thickness [1]. It involves temporary suturing of a radioactive ruthenium plaque to the external sclera to deliver a desired radiation dose to intra-ocular tumor. Radiation dose is prescribed by the treating ophthalmologist or radiation oncologist at a specific location, typically the tumor apex or scleral case [1, 2]; the medical physicist calculates the length of time the plaque remains sutured to the sclera, which is the treatment time required to deliver this dose. This treatment time is based on the tumor height and plaque calibration certificate, and generally assumes that the plaque is centered on the tumor.
This form of brachytherapy is used worldwide, and has demonstrated good rates of local control and eye preservation [3, 4]. Two commonly used 106Ru plaques are the circular plaques of 15.3 and 20.2 mm diameter (CCA and CCB, respectively; Eckert & Ziegler BEBIG GmbH, Berlin, Germany).
Plaque simulator™ (version 6.6.9, Eye Physics, LLC, Los Alamitos, CA, USA) is a software tool developed to model tumor-specific radiation dose distributions taking into account tumor location, thickness, shape, and plaque calibration data, to generate 3-dimensional models. Plaque simulator (PS) uses a patch source kernel, modelling doses in 300-1,000 kernels, and accounting for the scatter and attenuation, as the particles travel through the silver window, through an anisotropy term [5]. The uncertainty in the calculated dose is up to 2%; however, the modelling is based on plaque calibration certificates, which state a two sigma uncertainty in dose rates of 11%.
In addition to determining treatment time, it can generate dose-volume histograms (DVHs). DVHs plot relative dose as a function of tumor volume, and have been used to explore radiation dosing in both simulated and clinical scenarios [6-10]. For any radiation therapy involving 3D planning (such as intensity-modulated photon beam therapy), it has become widespread practice to report radiation dose delivered to specific percentages of tumor volume [11], with a commonly reported metric being D98% (the lowest radiation dose delivered to 98% of a given tumor volume). A recent retrospective case series accurately reconstructed prior 106Ru plaque placement and tumor characteristics in Plaque Simulator™, which revealed that specific tumor dose-volume percentages correlated with tumor control probability [12].
In certain clinical contexts, such as when anatomical structures make central plaque placement difficult, or when the surgeon wishes to reduce the dose to organs at risk by increasing the distance between these and the plaque, the plaque may be placed eccentrically [3, 13]. Previous studies have displayed the overall DVH trends of CCA and CCB plaques aligned to tumor center, anterior edge, or posterior edge [9, 10], but the effect of such eccentric plaque placement on specific dose volumes, such as D98%, remains unknown.
In this work, we aimed to systematically investigate the correlation between D98%, plaque eccentricity and the tumor height for two commonly used 106Ru plaques: CCA (15.3 mm diameter) and CCB (20.2 mm diameter), using Plaque Simulator™.
Material and methods
Plaque and tumor simulation
No ethics approval or consent was required for this study, as no patient data were utilized. The three-dimensional (3D) brachytherapy planning software plaque simulator (version 6.6.9, Eye Physics, LLC, Los Alamitos, CA, USA) was used for all simulations. Standard eye dimensions (24.0 mm equatorially × 26.2 mm antero-posteriorly) in PS were used.
Plaque simulator can model tumors that are dome-, peak-, and mushroom-shaped. For this work, only the most common shape, dome, was used. A high resolution grid setting was applied.
Two 106Ru plaques currently in use in our center were modelled using calibration certificates provided by the manufacturer (Eckert & Ziegler, BEBIG GmbH, Berlin, Germany). Within the software, a dome-shaped tumor was centered at 270° on the equatorial axis, with a basal diameter of 10 mm × 10 mm, or 7 mm × 7 mm. Tumor heights of two, three, four, five, and six millimeters were analyzed for each case.
Treatment time calculation
Treatment planning for the 10 mm diameter tumor was performed with CCB plaque, and for the 7 mm diameter tumor either CCA or CCB plaque. In each case, the plaque center was aligned with the tumor center on both coronal and sagittal axes. A plaque implantation time at one year following calibration certificate date was selected.
The normalized doses were calculated across the tumor volume, taking into account radioactive decay of ruthenium (half-life of 373.6 days) and modeled 3-dimensional dose distribution above the plaque. For each of the simulated tumors, treatment time was the time required to deliver prescription dose to 98% of the tumor volume (D98%). A scleral thickness of 1 mm was assumed and modelled in the software.
Eccentric plaque placement and D98% measurement
Alignment of posterior plaque edge to posterior tumor edge was performed using precise x axis coordinates in the software according to tumor diameter. Physical, rather than active edge of the plaque was aligned to tumor edge. The plaque was then moved anteriorly in regular 0.5 mm scleral cord length increments. Using the treatment time derived from a centered plaque, complete DVHs were generated and D98% values were determined. We assessed plaque eccentricity as the location of plaque edge in relation to the posterior tumor margin, which is how plaque placement location is typically established intra-operatively. Additionally, we established two safety margins from plaque edge to tumor edge: the minimum distances (mm) from the tumor edge to the plaque edge that D98% would be 95% and 90% of the centered D98% (SM95 and SM90), respectively.
Results
Treatment time and eccentric plaque placement
The treatment time required to deliver 100 Gy to 98% of the tumor volume varied across tumor diameters and plaque types (Table 1). These treatment times were used to generate eccentric plaque D98% values.
D98% measurement
For the 10 mm diameter tumor treated with the CCB plaque, a plaque off-set of 4 mm was required to reach the posterior tumor margin from the central plaque placement. For the 7 mm diameter tumor, the distance of plaque off-set from the central placement required to reach the posterior tumor margin was less for the CCA plaque (4 mm) than for the CCB plaque (6.5 mm).
Overall, D98% decreased as plaque eccentricity increased in each case (Figure 1). However, this trend was non-linear and did not follow a consistent pattern across tumor thicknesses for each discrete plaque model/tumor diameter iteration. Treated 2-4 mm thick tumors showed small increases in D98% when the plaque models were placed slightly eccentric to the tumor center. Greater placement eccentricities presented an inverse, non-linear relationship between plaque-tumor edge distance and D98%.
Fig. 1
D98% for eccentrical plaque placements, represented as plaque edge to tumor edge for A) 10 mm tumor treated with CCB plaque, B) 7 mm tumor treated with CCB plaque, and C) 7 mm tumor treated with CCA plaque. Different colored circles represent different tumor thicknesses (mm). D98% – radiation dose (Gy) delivered to 98% of tumor volume
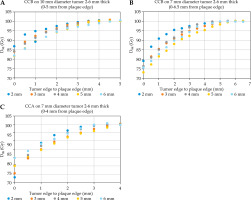
In general, increasing tumor thickness caused a larger overall decrease in D98% when plaque edge was closer to tumor edge, and a more rapid reduction in tumor D98%. For example, for a CCB plaque on 10 mm diameter tumor, tumor D98% dropped off further and more rapidly in 5 mm thick tumors than those that were 6 mm thick (Figure 1A). 5 mm thick tumors had the greatest overall D98% decrease for the CCB plaque in 7 mm and 10 mm diameter tumors (Figure 1A, B), and 2 mm thick tumors had the greatest overall drop off in tumor D98% for the CCA-treated 7 mm diameter tumors (Figure 1C). Interestingly, 2 mm thick tumors had the fastest rate of D98% decrease, but had the greatest and lowest overall decrease in D98% CCA and CCB plaques, respectively (Figure 1A-C).
Safety margins
The safety margins ranged from 1.1-3.7 mm (SM95) and 0.2-2.4 mm (SM90) (Figure 2). There was no consistent trend as seen in overall D98% values by eccentricity. Interestingly, for each given plaque mode/tumor diameter, all showed different patterns across tumor thicknesses. For each plaque/tumor combination, neither SM90 nor SM95 distances showed a consistent pattern across tumor thicknesses.
Fig. 2
Scleral cord distances from posterior plaque edge to posterior tumor edge, at which A) 90% of centered D98% was delivered (90 Gy), and B) 95% of centered D98% (d95) was delivered. Different colored circles represent different tumor thicknesses (mm). Different colored circles show different plaque model/tumor diameter cases. D98% – radiation dose (Gy) delivered to 98% of tumor volume, SM90 – safety margin of 90% of centered D98%, SM95 – safety margin of 95% of centered D98%
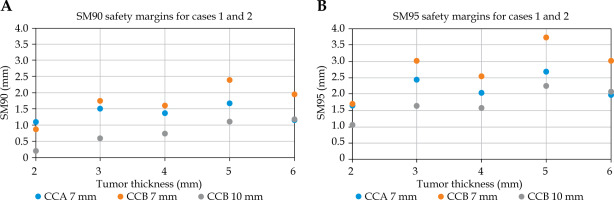
Discussion
The main finding of this study is that eccentric 106Ru plaque placement results in a reduction in the dose of radiation delivered to choroidal tumors by volume. In general, D98% dropped off more rapidly with decreasing tumor edge-plaque distance for thicker tumors. However, this trend was not uniform, highlighting the importance of 3-dimensional planning software, since tumor control probability depends on dose delivered to specific tumor volumes [12].
We believe the safety margins described in this work will be a useful tool for clinics, which do not routinely use 3-dimensional planning for 106Ru plaque brachytherapy.
Previous studies
When applied clinically, eccentric 106Ru plaque placement shows good preservation of vision and low rates of treatment failure, when additional measures are taken to ensure the accuracy of plaque placement [13]. However, as in the most published 106Ru brachytherapy studies, the reported dose delivery was the radiation dose to specific ocular coordinates (sclera, tumor base, and tumor apex) and the dose-rate, with the dose to tumor volume of the treated tumors not reported.
3-dimensional image-based planning for optimizing 106Ru plaque locations and treatment times has been demonstrated previously [14, 15]. Toxicities after ruthenium plaque brachytherapy include maculopathy, neuropathy, and retinopathy [16, 17]. Clinically, the ophthalmologist may use eccentric plaque placement to decrease the likelihood of these events occurring.
Previous work modelling eccentric plaque placements and tumor dose delivery has elucidated safety margins based on plaque radiation dose and isodose line shapes [18] for a range of tumors and tumor heights. Additional studies have made broad comparisons between overall DVHs of eccentric and centered CCA plaques for the treatment of 3 mm thick tumors [10, 19]. In contrast to our study, these investigations used Monte Carlo modelling and safety margins based on doses to specific ocular structures, namely the sclera, tumor base, and tumor apex. No previous work has, to our knowledge, used 3D treatment planning to examine a specific dose-volume percentage for eccentric 106Ru plaque placements across a range of tumor heights and diameters.
Limitations
D98% may not occur at the tumor apex (e.g., Figure 3A), and is dependent on the shape of isodose lines and tumor shape. The uncertainty in dose-rate values derived from the calibration certificate (2 sigma = 11%) limits the accuracy of calculated dose distributions. The uncertainty in our derived D98% values depends on several parameters, including dose-rate values derived from the calibration certificates (2 sigma ranges from 11% to 20% between plaque types), PS fit to the calibration data, dose calculation, and extraction of D98% value themselves from generated DVH.
Fig. 3
Isodose lines for CCA plaque for tumors of varying thicknesses and eccentricities to posterior plaque edge: A) 2 mm thick tumor, 0 mm eccentric to posterior tumor edge; B) 2 mm thick tumor, 2 mm eccentric to plaque edge; C) 5 mm thick tumor, 0 mm eccentric to plaque edge; D) 5 mm thick tumor, 2 mm eccentric to plaque edge. Different colored lines represent locations where the same radiation dose (Gy) is delivered
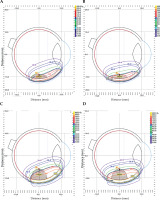
This work determines safety margins for one CCA and one CCB plaque model only, and while plaques of the same model are similar, there are dosimetric differences between plaques of the same model, which may affect the proposed safety margins. Similarly, the safety margin values generated were for a unidirectional off-set only, and the dose fall-off away from the plaque central axis was not entirely symmetrical. The heterogeneity of radiation emission distribution across 106Ru plaque surfaces has been described in previous works [9, 19, 20].
In addition to eccentric plaque placement, plaque tilt would also affect the dosimetric coverage of the tumor [21], which was acknowledged in recent 106Ru brachytherapy investigation [22]. However, plaque tilt was not investigated in the present work.
Plaque simulator is not FDA/CE-certified; nevertheless, it is the only available image-based planning system for ophthalmic plaques.
Future directions
Future intended work includes the determination of safety margins for other tumor shapes and plaque types (other than CCA and CCB), evaluating the applicability of our calculated safety margins to different CCA and CCB models, and the effect of tumor off-set on dose delivery to proximal critical organs [15]. Only dome-shaped tumors were modelled in this work; exploring safety margins for other tumor shapes (e.g., mushroom and peak) would be valuable.
Conclusions
D98% in tumors treated with 106Ru plaque brachytherapy decreases as plaque eccentricity increases. However, D98% changes according to tumor shape, thickness, and plaque model. Treatment times assuming central plaque placement should be modified for eccentric treatments in order to optimize the expected tumor control.