Introduction
Sepsis, a systemic inflammatory response syndrome (SIRS) stimulated by infection, is usually complicated by acute kidney injury (AKI) [1-3], which contributes to a high mortality of 40-60% [4]. Lipopolysaccharide (LPS), an ingredient of gram-negative bacteria, has been commonly applied to establish cell models of sepsis-induced AKI [5]. It was documented that LPS-treated renal tubular epithelial cells could overproduce inflammatory cytokines, such as interleukin (IL)-6 and tumor necrosis factor α (TNF-α), thereby badly impairing kidney function [6]. There is also a consensus that excessive apoptosis of renal tubular epithelial cells, driven by LPS, fosters deterioration of sepsis-relevant AKI [1, 7, 8]. Hence, in order to improve treatments for sepsis-induced AKI, it was of significance to curb dysregulated cell apoptosis and over-production of inflammatory cytokines in the kidney.
Resveratrol (chemical name: 3,4,5-trihydroxy-diphenyl), a polyphenolic compound, was initially extracted from rhizome of Veratrum album by a Japanese scientist named Takaoka. This natural antibiotic not merely fought against bacteria-/fungus-induced infections, but also prevented onset of cancers, cardiovascular diseases and inflammatory disorders [9-11]. Of note, it was reported that resveratrol attenuated inflammation in AKI by activation of nuclear factor-erythroid-2-related factor 2 (Nrf2) signaling [12] and suppression of nuclear factor-κB (NF-κB) signaling [13], which implied that resveratrol was likely to be involved in the etiology of sepsis-relevant AKI by regulating molecular networks.
A myriad of evidence has demonstrated that resveratrol modulates disease progression by altering expression of miRNAs [14-16]. For instance, levels of miR-663, miR-146a and miR-21, crucial biomarkers of sepsis onset [1, 17, 18], were up-regulated in the resveratrol-treated SW480 cell line [19]. Notably, lncRNAs were documented to sponge miRNAs, so as to reduce inhibition of miRNAs on disease-related genes. Taking sepsis for example, lncRNA SNHG16 was found to up-regulate TLR4 expression in LPS-treated RAW264.7 cells by binding to miR-15a/16 [20]. However, lncRNAs that were involved in resveratrol’s ameliorating sepsis-induced AKI have rarely been investigated.
LncRNA MALAT1, initially speculated as a tumor biomarker [21], revealed positive correlations with serum levels of TNF-α, IL-1β, IL-6, IL-10, IL-17 and interferon γ (IFN-γ) in septic rats [22], and an incremental level of MALAT1 was suggestive of unfavorable survival among sepsis patients [23]. MALAT1 was also reported to exacerbate sepsis-triggered cardiac inflammation by interacting with miR-125b [22]; nonetheless, little was known about the significance of MALAT1 in sepsis-derived AKI. Interestingly, several MALAT1-sponged miRNAs were documented to participate in AKI etiology; for example, miR-204, down-regulated by MALAT1 in lung adenocarcinoma [24], was able to relieve sepsis-caused AKI [25]. Moreover, miR-205, sponged by MALAT1 in Parkinson’s disease (PD) [26], reduced the likelihood of AKI onset among sepsis patients, and patients carrying lowly-expressed miR-205 tended to enjoy a longer life span than those with high miR-205 expression [27]. Although MALAT1 might be involved in sepsis-derived AKI by regulation of miRNAs, whether it was implicated in the protective effect of resveratrol against sepsis-caused AKI remained uncertain.
Therefore, this study was intended to determine whether resveratrol attenuated sepsis-triggered AKI by down-regulating MALAT1, which might provide new treatment strategies for sepsis patients.
Material and methods
Establishment of sepsis models in rats
Detection of cytokines in serum of rats
120 male specific-pathogen-free male Sprague-Dawley (SD) rats, aged 13-15 weeks old and weighing 200-250 g, were provided by the experimental animal center of No. 1 People’s Hospital (First Affiliated Hospital of Xiang Nan University) (Hu’nan province, China). These rats were randomly divided into a blank control group (n = 20), a Sham operation group (Sham group, n = 20), a cecal ligation and puncture (CLP) group (Sep group, n = 40) and a resveratrol group (Res group, n = 40), and they were fasted for 12 h prior to surgery, with free access to water. The abdomen of rats in the Sham group was closed immediately after laparotomy, while the ceca of rats in the CLP group and Res group were ligated and punctured before abdominal closure. In the wake of successful modeling, rats of the Res group were injected with 0.3 ml/100 g Res solution (Catalog number: 13065-54-8, purity ≥ 99%, Hubei shengtian henchuang pharmaceutical corporation, China) from the tail vein, and rats of the other groups were injected with equal amounts of normal saline. Around 12 h after surgery, 5 ml of blood was extracted from the abdominal aorta of each rat, and the blood samples were centrifuged at 5000 r/min for 15 min to determine serum levels of cytokines and MALAT1: 1) serum levels of NGAL, KIM-1, TNF-α, IL-1β and IL-6 were determined with corresponding ELISA kits (BD, USA), 2) serum levels of Scr, blood urea nitrogen (BUN) and Scys C were measured using a MedLab automated biomedical analyzer (Nanjing medease science and technology corporation, China), and 3) serum level of MALAT1 was detected via PCR as detailed below.
Tracking survival of model rats
Another population of rats (n = 120) under the same treatments, including a control group (n = 20), a Sham group (n = 20), a Sep group (n = 40) and a Res group (n = 40), was tracked for survival, and blood samples were taken immediately after death of the rats or until end of the observation period.
All animal experiments in this study were conducted according to the requirements of Care and Use of Experimental Animals of the US National Institutes of Health (Bethesda, MD, USA), and this program has obtained approval from the Animal Care Committee of No. 1 People’s Hospital (First Affiliated Hospital of Xiang Nan University) (Hu’nan province, China).
Cell culture
Human kidney proximal tubular epithelial cell 2 (HK2) cell line, purchased from American Type Culture Collection (ATCC, USA), was inoculated in minimum Eagle’s medium (MEM), which contained 10% fetal bovine serum (FBS), 1% penicillin-streptomycin and 1% non-essential amino acids (NEAA), under an atmosphere of 37°C and 5% CO2.
Cell transfection
HK2 cells seeded within 6-well plates were cultured for 24 h, until cell confluence occurred at 90%. Then si-MALAT1, si-NC, pcDNA3.1 and pcDNA3.1-MALAT1, all purchased from Ribobio (China), were separately transfected into HK2 cells for 48 h, as per guidance of the Lipofectamine 2000 transfection kit (Invitrogen, USA).
Cell treatment with LPS and resveratrol
HK2 cells were treated through 3 approaches: 1) LPS treatment: addition of 10 µg/ml LPS (Sigma, USA), 2) PBS treatment: addition of equal volumes of PBS, and 3) Res + LPS treatment: supplementation of 200 µmol/l resveratrol (Enzo life sciences, USA) for 2 h and then addition of 10 µg/ml LPS. After 6-h cell culture, supernatant of HK2 cells was collected after centrifugation, and then they were stored at –80°C in the refrigerator.
Real-time polymerase chain reaction (PCR)
After extraction from blood samples and HK2 cells with the TRIzol method (TaKaRa, Japan), density and purity of total RNAs were measured by an ultraviolet spectrometer (HITACHI, Japan). Then cDNAs of MALAT1 were transformed from RNAs according to the manual of the Prime-Script RT reagent kit (TaKaRa, Japan), and cDNAs of miR-205 were synthesized in the light of specifications of the One Step miRNA PrimeScript cDNA synthesis kit (TaKaRa, Japan). Using primers of target genes (Table 1), the cDNAs were amplified in line with instructions of the SYBR Premix Ex Taq II kit (TaKaRa, Japan). Relative expression levels of genes were determined using the 2-△△Ct method, and gene levels in the control group were normalized to 1.
Table 1
Primers for genes
CCK8 assay to assess proliferation of HK2 cells
HK2 cells of the logarithmic growth phase were inoculated into 96-well plates at the density of 5 × 104/ml for 48 h. Subsequently, HK2 cells of each well were treated with 10 µl of CCK8 solution (Beyotime, USA) for 4 h, and the absorbance value (A) of cell samples was measured at the wavelength of 570 nm using a microplate reader (model: BIORAD-550, Bio-Rad, USA).
Determination of cytokines secreted by HK2 cells
Levels of IL-1β, IL-6 and TNF-α in the supernatant of HK2 cells were detected with corresponding ELISA kits (BD, USA).
Statistical analyses
All the data were statistically analyzed by means of SPSS13.0 statistical software. Quantitative data, all presented as mean ± standard deviation (SD), were compared with Student’s t test or one-way analysis of variance (ANOVA), and comparison of enumeration data was conducted with the χ2 test. Pearson correlation was implemented to analyze correlations between serum MALAT1 level and biomarker levels, and Kaplan-Meier curves were plotted to estimate the association of serum MALAT1 level with survival of rats. P value < 0.05 was deemed statistically significant.
Results
Resveratrol affected serum levels of MALAT1, as well as indicators of inflammatory response, kidney function and AKI, in the septic rat model
Rats of the Sep group had significantly higher serum levels of TNF-α, IL-1β and IL-6 than rats of the Sham group (all p < 0.001), and levels of the cytokines were significantly lower in the Sep + Res group as compared with the Sep group (all p < 0.001) (Fig. 1A). In addition, Scr, BUN and Scys C levels, suggestive of renal function [29], were markedly raised in septic model rats as compared to rats of the Sham group (all p < 0.001), yet administration of resveratrol (i.e. Rep group) significantly reduced Scr, BUN and Scys C levels in comparison to the Sep group (all p < 0.001) (Fig. 1B). When compared with rats of the Sham group, NGAL and KIM-1 levels, pivotal indicators of AKI [30], were increased in septic model rats (all p < 0.001), while resveratrol treatment (i.e. Rep group) mitigated AKI injuries in septic rats (all p < 0.001) (Fig. 1C). Moreover, serum level of MALAT1 was raised in model rats of Sep group (all p < 0.001), but it fell markedly in the Sep + Res group as compared with Sep group (all p < 0.001) (Fig. 1D).
MALAT1 level was correlated with biomarker levels indicative of inflammation, kidney function and AKI in serum of resveratrol-treated septic model rats
MALAT1 level was positively correlated with serum levels of IL-1β (Fig. 2A), IL-6 (Fig. 2B) and TNF-α (Fig. 2C) in model rats of sepsis group (IL-1β: rs = 0.358, p < 0.001; IL-6: rs = 0.481, p < 0.001; TNF-α: r2 = 0.349, p < 0.001) and Sep + Res group (IL-1β: r2 = 0.576, p < 0.001; IL-6: r2 = 0.325, p < 0.001; TNF-α: r2 = 0.596, p < 0.001). There were also pronounced correlations between MALAT1 level and levels of Scr (Fig. 3A), BUN (Fig. 3B) and Scys C (Fig. 3C) among rats of sepsis group (Scr: r2 = 0.497, p < 0.001; BUN: r2 = 0.348, p < 0.001; Scys C: r2 = 0.228, p = 0.002) and rats of Sep + Res group (Scr: r2 = 0.548, p < 0.001; BUN: r2 = 0.615, p < 0.001; Scys C: r2 = 0.401, p < 0.001). Furthermore, MALAT1 level rose significantly as NGAL (Fig. 4A) and KIM-1 (Fig. 4B) levels were elevated in model rats of the sepsis group (NGAL: rs = 0.421, p < 0.001; KIM-1: rs = 0.484, p < 0.001) and Sep + Res group (NGAL: rs = 0.613, p < 0.001; KIM-1: rs = 0.577, p < 0.001). Nonetheless, hardly any significance was discerned in the correlation between serum level of MALAT1 and serum level of IL-1β (p = 0.302), IL-6 (p = 0.827), TNF-α (p = 0.128), Scr (p = 0.881), BUN (p = 0.711), Scys C (p = 0.758), NGAL (p = 0.492) or KIM-1 (p = 0.402) (Figs. 2-4).
Fig. 2
LncRNA MALAT1 level was correlated with serum levels of IL-1β (A), IL-6 (B) and TNF-α (C) among rats of control group combined with Sham group combined, Sep group and Sep + Res group
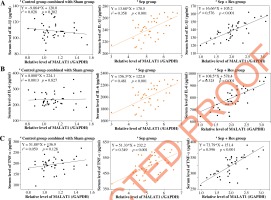
MALAT1 level was predictive of survival among septic model rats
Among 120 model rats whose survival status was tracked, rats of the Sep group demonstrated a higher MALAT1 level than rats of the Sham group (p < 0.001), while rats of the Rep group had a much lower average MALAT1 level than rats of the Sep group (p < 0.001) (Fig. 5A). Furthermore, survival of rats in the sepsis group was quite unfavorable as compared with rats of the Sham group and control group (p < 0.001), and septic rats under resveratrol treatment (i.e. Sep + Res group) demonstrated longer survival than septic model rats (p < 0.05) (Fig. 5B). With median MALAT1 level (i.e. 7.07) as the cut-off point, we found that septic rats with a high (> median) MALAT1 level revealed shorter survival than rats with a low (≤ median) MALAT1 level (p < 0.01) (Fig. 5C). Also using median MALAT1 level as the dividing point, rats of the Sep + Res group enjoyed more ideal survival if they had a low (≤ 2.56) MALAT1 level, with the high-level (> 2.56) MALAT1 group as the reference (p < 0.001) (Fig. 5D).
Fig. 5
Association of lncRNA MALAT1 level with survival of model rats under different treatments. A) Serum level of lncRNA MALAT1 was determined among model rats of control group, Sham group, Sep group and Sep + Res group. ***p < 0.001. B) Survival rates of model rats were compared among control group, Sham group, Sep group and Sep + Res group. C) Survival rates of model rats in the sepsis group were compared between high-level MALAT1 group and low-level MALAT1 group. D) Survival of model rats in the Sep + Res group was compared between high-level MALAT1 group and low-level MALAT1
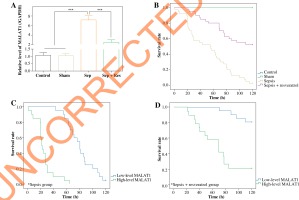
group Resveratrol diminished viability and cytokine release of HK2 cells by down-regulating MALAT1 expression
MALAT1 expression increased markedly in LPS-treated HK2 cells (p < 0.001), yet resveratrol treatment (i.e. LPS + resveratrol group) gave rise to a sharp decrease of MALAT1 expression in septic cell models (p < 0.001) (Fig. 6A). Moreover, MALAT1 expression was up-regulated in HK2 cells transfected by pcDNA3.1- MALAT1 (p < 0.001), yet was down-regulated as MALAT1 was silenced (p < 0.001) (Fig. 6B).
Fig. 6
Resveratrol reduced proliferation and inflammatory response of HK2 cells by regulating lncRNA MALAT1 and miR-205. A) MALAT1 expression was determined in HK2 cells of PBS group, LPS group and LPS + Res group. ***p < 0.001. B) MALAT1 expression in HK2 cells was altered after transfection of si-MALAT1 and pcDNA3.1- MALAT1. ***p < 0.001. C) Viability of HK2 cell line was determined after treatments of PBS, LPS, LPS + Res, LPS + si-MALAT1 and LPS + Res + pcDNA3.1-MALAT1. ***p < 0.001 in comparison to PBS group; ###p < 0.001 in comparison to LPS group; @@@p < 0.001 in comparison to LPS + Res group. D-F) TNF-α (D), IL-1β (E) and IL-6 (F) levels were compared among PBS group, LPS group, LPS + Res group, LPS + si-MALAT1 group and LPS + Res + pcDNA3.1-MALAT1 group. ***p < 0.001 in comparison to PBS group; ###p < 0.001 in comparison to LPS group; @@@p < 0.001 in comparison to LPS + Res group. G) MiR-205 expression in HK2 cells was measured after transfection of si-MALAT1 and pcDNA3.1-MALAT1. ***p < 0.001. H) MiR-205 expression in HK2 cells was compared among PBS group, LPS group, LPS + Res group, LPS + si-MALAT1 group and LPS + Res + pcDNA3.1-MALAT1 group. ***p < 0.001 in comparison to PBS group; ###p < 0.001 in comparison to LPS group; @@@p < 0.001 in comparison to LPS + Res group
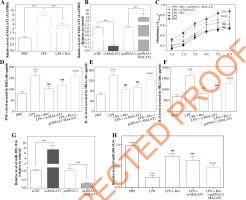
Viability of HK2 cells was strengthened after stimulation of LPS, when compared with the control group (p < 0.001) (Fig. 6C). Both resveratrol and si-MALAT1 were capable of undermining viability of LPS-treated HK2 cells (p < 0.001), although resveratrol played a stronger restraining role than si-MALAT1 (p < 0.001). Also the suppressive effect of resveratrol (i.e. LPS + resveratrol group) on viability of LPS-treated HK2 cells could be alleviated by pcDNA3.1-MALAT1 (i.e. LPS + resveratrol + pcDNA3.1-MALAT1 group) (p < 0.001).
In addition, TNF-α, IL-1β and IL-6 levels were dramatically up-regulated in supernatants of HK2 cells after induction of LPS (p < 0.001) (Fig. 6D-F). Nevertheless, the cytokine levels were decreased in HK2 cells of the LPS + resveratrol group and LPS + si-MALAT1 group relative to HK2 cells of the LPS group (p < 0.001). Moreover, TNF-α, IL-1β and IL-6 levels in the LPS + resveratrol group were up-regulated by extra transfection of pcDNA3.1-MALAT1 (i.e. resveratrol + LPS + pcDNA3.1-MALAT1 group) (p < 0.001).
Resveratrol and MALAT1 impacted miR-205 expression in HK2 cells
MiR-205 expression in HK2 cells was raised after transfection of si-MALAT1 (p < 0.001), and was reduced by pcDNA3.1-MALAT1 (p < 0.001) (Fig. 6G). Moreover, miR-205 expression exhibited a drop after LPS treatment (p < 0.001), while resveratrol (i.e. resveratrol + LPS group) and si-MALAT1 (i.e. si-MALAT1 + LPS group) both up-regulated miR-205 expression in LPS-treated HK2 cells (p < 0.001) (Fig. 6H). Moreover, transfection of pcDNA3.1-MALAT1 (i.e. resveratrol + LPS + pcDNA3.1-MALAT1 group) led to increased miR-205 expression in resveratrol + LPS-treated HK2 cells (p < 0.001) (Fig. 6H).
Discussion
Up to 19% of sepsis patients simultaneously develop AKI [31], and a large number of them often miss the golden timing for treatment [6], owing to the absence of sensitive diagnostic biomarkers [32]. Therefore, to benefit patients with sepsis-induced AKI, investigating its underlying etiology is necessary.
It was noteworthy that the inflammatory cascade was intensified during septic progression, which could severely damage renal function [33]. In this investigation, we also discovered that inflammation was abnormally magnified, along with destroyed renal function, in CLP-induced septic model rats; however, this physiological change was relieved after resveratrol injection (Fig. 1). Multifold signaling pathways have been documented to explain the protective role of resveratrol against inflammation-caused damage [34]. For instance, TLR2/NF-κB signaling and mitogen-activated protein kinase (MAPK) signaling, both motivated by LPS, could be deactivated by resveratrol treatment, so that exacerbation of inflammatory diseases was prevented [35, 36]. Expanding perceptions of this aspect, this investigation newly revealed that lncRNA MALAT1 which was up-regulated in CLP-induced septic model rats was, to some extent, normalized after resveratrol treatment (Fig. 1D). Furthermore, given that variation of MALAT1 expression was reflective of renal function (Figs. 3-4), inflammatory response (Fig. 2) and survival status (Fig. 5) of septic model rats, resveratrol was likely to ameliorate sepsis-relevant AKI by inhibiting MALAT1-led signaling pathways.
LncRNAs have displayed huge potential with respect to averting inflammatory injuries through regulating mitochondrial division, pro-inflammatory cytokines, calcium ion transport and NF-κB signaling [37, 38]. For example, linc 0206, linc 3995, linc7190 and linc 7705 powerfully down-regulated TNF-α and IL-6 expression in Pam3CSK4-stimulated THP1 macrophages [39], while expressions of E-selectin, vascular cell adhesion molecule-1 (VCAM-1), IL-8 and IL-6 were negatively modulated by lncRNA human interleukin 7 receptor (lnc-IL7R) in LPS-treated THP1 macrophages [40]. Concerning MALAT1 studied here, which was over-expressed in LPS-treated macrophages [41], its low expression could protect myocardia from inflammatory damage by sponging miR-26a and down-regulating TLR4 [42], and ERK/MAPK signaling, pivotal to AKI development [43], might be activated by MALAT1 [44]. These proofs all supported the role of MALAT1-led signaling pathways in protecting against sepsis-relevant inflammation and AKI, providing a molecular approach that resveratrol depended on to fight against sepsis-induced AKI.
Additionally, cell models were built to confirm the association of the resveratrol/MALAT1 axis with sepsis-relevant inflammation (Fig. 6). We observed that HK-2 cells treated with LPS released higher levels of IL-6, IL-1β and TNF-α than the control group (Fig. 6D-F), indicating that sepsis-induced AKI cell models were successfully established [5, 45]. Furthermore, resveratrol was discovered to repress viability (Fig. 6C) and decrease IL-6, IL-1β and TNF-α production in LPS-treated HK2 cells (Fig. 6D-F), implying in vitro protection of resveratrol against inflammation injury. More interestingly, MALAT1 level varied when HK2 cells was managed by resveratrol (Fig. 6A), and si-MALAT1, similar to resveratrol, also protected HK2 cells from being destroyed by LPS (Fig. 6D-F). Additionally, pcDNA3.1-MALAT1 could, to some extent, prohibit the effect of resveratrol on viability and cytokine production of HK2 cells (Fig. 6C-F), which all suggested that MALAT1 was subjected to in-vitro regulation of resveratrol. Furthermore, miR-205 expression, previously reported to prevent sepsis-triggered AKI [27], was raised by resveratrol and si-MALAT1 (Fig. 7G, H), providing a hint that the MALAT1/miR-205 axis was probably involved in the contribution of resveratrol to AKI in sepsis.
In conclusion, intake of resveratrol was conducive to reliving inflammation and AKI in sepsis by suppressing the MALAT1/miR-205 axis. However, several pitfalls could limit the reliability of this conclusion. Firstly, now we were unable to recruit AKI patients with resveratrol treatment, so the clinical efficacy of resveratrol on AKI in sepsis could not be visually presented. Secondly, though regulation of MALAT1 on miR-205 was disclosed, how this modulation was formed was not investigated. Thirdly, MALAT1 downstream pathways, which were alterable by resveratrol, were not studied in depth. The points raised above all demand solid proof in future.