Introduction
Type 1 diabetes (T1DM) is one of the most common autoimmune endocrine and metabolic diseases in the world [1]. According to the currently adopted model of the pathogenesis of T1DM, proposed in 1986 by George Eisenbarth, 3 components are crucial for the development of T1DM: genetic and environmental factors, and the impact of the patient's immune system [2, 3]. Among the genetic factors, the HLA system on chromosome 6 is of the greatest importance. More than 40 loci have been associated with a predisposition to T1DM [4]. The population risk increases 3-fold when the father of the child suffers from T1D and 2-fold when the mother suffers from diabetes mellitus. In the case of sick siblings, the risk increases to 4% by age 20 years, and up to 9.6% by age 60 years [5].
Environmental factors are thought to accelerate the process of self-destruction of pancreatic β cells, but they are probably not responsible for the initiation of autoimmunity [6, 7]. Among environmental factors, the protective role of breastfeeding, vitamin D3 supplementation, and the dominance of Bacteroides bacteria in the intestinal microbiota are emphasized [8–10]. The environmental factors accelerating/initiating the autoimmune reaction against one's own tissues are infection with enteroviruses, including Coxsackie B4 viruses [11–13]. Viral peptides, similar in structure to pancreatic β cell proteins, stimulate the host's B and T lymphocytes, which has been termed molecular mimicry [14].
An extensive review of the available literature on the association of group B coxsackie virus (CV-B) infection with the role of NK cells in the pathogenesis of T1DM was performed by Nekoua et al. [15]. The authors described how CV-B, by reducing HLA class I expression, avoids recognition and attack by T lymphocytes, while at the same time, B cells infected with CV-B virus are destroyed by NK cells. As a result, the process of destroying these infected cells is ineffective, a prolonged inflammatory reaction occurs, and antigens are released from apoptotic B cells that contribute to the process of autoimmunity against pancreatic islets. This is also confirmed by the results of the original work from this centre [15, 16].
However, in many studies in animal models and in a group of patients, it was not possible to identify the factors directly responsible for the development of T1DM [17, 18]. Typically, early in life, due to unknown factors, there is a loss of immune tolerance, the release of self-antigens, and a cascade of self-destruction [19]. The course of the autoimmune reaction in the pathogenesis of T1DM is shown in Figure 1.
Figure 1
Cascade of destruction in the pathogenesis of T1DM. In the first phase, viral infection, activation of NK cells, macrophages, and Teff cells lead to apoptosis of pancreatic b cells. The b cell antigens released during apoptosis are presented to the APCs. Phase 2 takes place in the draining lymph nodes, where the APC cell interacts with the Teff cell, prompting the B cells to produce anti-islets antibodies. At the same time, information is transferred to Treg cells, which, together with NKT-like cells, are responsible, in phase 3 in the pancreas, for inhibiting the immune response of NK, Teff, and APC cells. Breaking the immune balance leads to a cascade of destruction and underlies the pathogenesis of T1DM
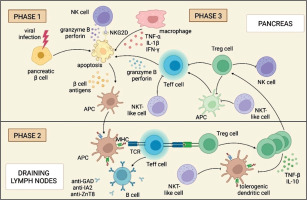
The role of NK cells in the development of T1DM is probably related to the ability to kill target cells and to interact with antigen presenting cells (APCs) and T cells [20–24]. NK cells were identified as one of the major subpopulations in islet infiltration in an animal model of insulitis [25–27], and blocking NK cell activation in the infiltrate resulted in inhibition of disease progression [28, 29]. NK cells also have the ability to shift the immune response towards the Th-2-dependent mechanism in the TGF-β-dependent mechanism, which is why they are able to have a protective effect on pancreatic β cells [30]. It has been suggested that the NK cell population could become a potential therapeutic target in T1DM.The aim of the study was to evaluate the population of NK cells and antibodies against pancreatic β cells in children with T1DM and their healthy siblings in comparison with children from families not burdened with autoimmune diseases.
Material and methods
Study group
The first group of respondents, comprising 76 people, 46 boys and 30 girls (average age 12 years), were children with T1DM diagnosed and treated at the Department of Paediatric Endocrinology and Diabetology in 2014–2015. Patients with newly diagnosed T1DM were enrolled in the research after recovery from ketosis and achieving relative glycaemic control.
The second group of respondents, comprising 101 people, 50 boys and 51 girls (average age 11 years and 4 months), were healthy siblings of the studied children with type 1 diabetes, and without symptoms of diabetes and glucose metabolism disorders.
The age-matched control group consisted of 30 children, 21 boys and 9 girls (average age 11 years and 2 months), hospitalized in the Department of Paediatric Endocrinology and Diabetology of the Medical University of Lublin in 2014–2015, during a control visit after successful treatment of obesity, in whom physical examination and laboratory tests excluded glucose metabolism disorders and autoimmune diseases.
All persons during the study and in the month preceding the study did not show any signs of infection, did not take medications affecting the immune system, and did not have any blood transfusions in the 3 months prior to the study.
Ethical standards
Informed consent to participate in the study was obtained from legal guardians and patients above the age of 16 years before entering the study. The clinical trial protocol was approved by the Bioethics Committee at the Medical University of Lublin, No. KE-0254/153/2012.
Determination of the antibody level in serum
The material for the research was peripheral blood taken from the peripheral vein for the determination of the level of antibodies and the isolation of NK cells.
The material was centrifuged for 10 min at an acceleration of 300 g to obtain serum for the determination of the antibody level. The serum obtained in this way was transported to the hospital laboratory (ALAB). Anti-glutamic acid decarboxylase antibodies (anti-GAD) (EUROIMMUN, Germany), anti-tyrosine phosphatase antibodies (anti-IA2) (EUROIMMUN, Germany), and anti-zinc transporter 8 antibodies (anti-ZnT8A) (Demeditec, Germany) were evaluated by ELISA. Determinations were performed using materials and reagents according to the procedures included by the manufacturer with each kit. To read the results, an automatic VICTOR3 reader (Perkin Elmer, USA) and the WorkOut2 2.0 computer program cooperating with the reader were used.
To assess the percentage of circulated NK cells, freshly collected whole blood was immediately transported to the Department of Clinical Immunology of the Medical University of Lublin. The NK cell population was determined using a FACSCalibur flow cytometer (Becton Dickinson, USA). To analyse the results and their graphic presentation, CellQuest Pro computer software (Becton Dickinson, USA) was used.
200 µl of freshly drawn EDTA whole blood was used to determine the NK cell population. The Simultest CD3/CD16+CD56 Reagent Becton Dickinson kit was used. Due to the combination of anti-CD56, CD16, and CD3 antibodies, it was possible to isolate the NK cell population from mononuclear cells in peripheral whole blood. CD3 monoclonal antibodies were marked with fluorescein (FITC), and anti-CD16 and anti-CD56 were labelled with phycoeritin (PE). The test tube was incubated with 50 µl of whole blood with 5 µl of marked antibodies for 20 min at room temperature in the dark. The red blood cells were then lysed 10 times with BD FACS™ Lysing Solution. One part of the starting fluid (10) was used with 9 parts distilled water, and then incubated for 15 min at room temperature in the dark. The slide was centrifuged for 5 min at an acceleration of 700 g at room temperature. After decanting the supernatant, the slide was washed twice in PBS solution without calcium and magnesium. The cells prepared in this way were subjected to cytometric analysis.
The values of the analysed parameters measured on the nominal scale were characterized by the number and percentage, while those measured on the quotient scale were characterized by means of the mean, median, standard deviation, and range of variation.
The distribution of the investigated quantitative parameters was assessed using the Shapiro-Wilk test. Student’s t-test with a grouping variable or the Mann-Whitney U test was used to detect the existence of differences between the 2 analysed groups for measurable features.
The non-parametric test for Spearman’s rank correlation coefficient or the test for Pearson’s correlation coefficient were used to determine the relationship between the investigated quantitative features.
To assess the differences between the analysed groups for nominal characteristics, the test of independence χ2 or χ2 with Yates’ correction was used if any of the expected numbers was less than 5.
The diagnostic usefulness of the test was assessed taking into account the number of true positive (TP), false positive (FP), false negative (FN), and true negative (TN) results. In addition, the basic measures of test accuracy were used: sensitivity and specificity.
Statistical analysis
Because the values of the diagnostic test were continuous, predictive values were calculated for the best at differentiating patients with normal and abnormal results of the optimal threshold values. In the analysis, the changes in the sensitivity and specificity of the parameters were presented in the form of a ROC curve. The assessment of the area under the ROC curve (AUROC) determines the diagnostic usefulness of a given parameter. The larger the area under the ROC curve, the better the diagnostic efficiency of a given laboratory parameter.
A 5% inference error and the related significance level p < 0.05 were assumed, indicating the existence of statistically significant relationships or differences. The database of analysed data and statistical analyses were performed using Statistica 12.0 computer software (StatSoft, Poland).
The age of the children with T1DM was in the range from 2 to 22 years, the age of their siblings was in the range from 7 months to 27 years, and the age of the control group was in the range from 6 to 14 years. In the group of children with T1DM and in the control group, children in the age range of 8–18 years predominated (i.e. in the group of children with T1DM: 73.68%, n = 56; in the control group: 80%, n = 24). The most numerous age range in the group of siblings of children with diabetes were patients under 8 years old (42.57%, n = 43). In all groups of respondents, the least numerous group comprised young adults aged > 18 years. The highest average age was found in the group of children with T1DM, and the lowest was found in the group of their siblings. In the control group, the average age was 11.19 years. There were no significant age differences between girls and boys in all study groups (Student’s t-test for children with diabetes t = 0.75; p = 0.45; for the siblings group t = 0.45; p = 0.66; and for the control group t = 1.19; p = 0.58). The study groups did not differ statistically significantly in terms of age.
Results
The mean percentage of NK cells in children with T1DM (10.59 ±5.37) and in the siblings group (11.93 ±5.62) was statistically significantly reduced in comparison with the control group (14.89 ±7.78) consecutively (Student’s t-test: t = –3.24; df = 103; p = 0.002) (Student’s t-test: t = –2.30; df = 128; p = 0.02). There was no statistically significant difference in the percentage of NK cells between the group of children with T1DM and their siblings (Student’s t-test: t = –1.59; df = 173; p = 0.11) (Table I).
Table I
NK cells as the percentage of lymphocytes in children with T1DM, their siblings, and in the control group without autoimmune diseases
In the control group and in the group of siblings of the patients with T1DM, the younger the child, the lower percentage of NK cells noticed, and the older the child, the higher percentage of NK cells (on average 0.28/year). This relationship was statistically significant (Test for Pearson’s correlation coefficient t = 3.41; p = 0.0009; r = 0.33). A similar relationship was not noticed in the group of children with T1DM. The dependence of the number of NK cells on the age of the child in the group of siblings is shown in Figure 2.
Figure 2
The influence of age on the percentage of NK cells in the group of siblings of children with T1DM
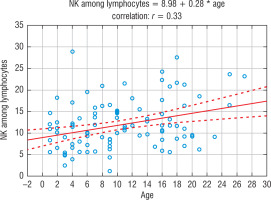
The characteristics of the values of the analysed antibodies in each study group and the significance between the groups are presented in Table II.
Table II
The concentration of autoantibodies against pancreatic b cells in the studied groups and the significance of differences between the groups
The concentration of anti-IA2 and anti-Znt8 antibodies was statistically significantly higher in the siblings group in comparison with the control group (anti-IA2 p = 0.0000001; anti-ZnT8 p = 0.00001), and the concentration of anti-GAD antibodies was comparable in both groups.
Among all the examined children, the most common antibody was anti-GAD (27.54%, n = 57), followed by anti-ZnT8 (26.57%, n = 55), and least often anti-IA2 (23.67%, n = 49). These 3 tested antibodies were noticed in 26 children (12.56%), including one child (from the group of siblings) without diagnosed T1DM. Among the 2 antibodies directed against pancreatic β cells coexisting in serum, the most frequently reported were simultaneously elevated anti-GAD level with anti-ZnT8 (5.31%, n = 11), followed by anti-GAD with anti-IA2 (4.83%, n = 10), and least frequently anti-IA2 with anti-GAD (2.42%, n = 5). In the siblings group, 2 antibodies (1.98%) were found in 2 examined children, including one person (0.99%) with anti-GAD and anti-IA2, and one patient (0.99%) with anti-IA2 and anti-ZnT8.
In the group of children with T1DM, there was a positive correlation between the reduced percentage of NK cells and the concomitance of anti-GAD and anti-ZnT8 antibodies (Mann-Whitney U test Z = –2.02; p = 0.04). There was no similar relationship in the group of siblings.
A reduced percentage of NK cells among lymphocytes can be useful for identifying people who are sick or predisposed to developing T1DM. Using the ROC curve method, it was noted that the percentage of NK cells differentiates children with T1DM with average effectiveness according to AUC values, with low sensitivity (55%) and specificity (60%). The relevant ROC curve is shown in Figure 3.
Discussion
The autoimmune basis of T1DM has been unequivocally confirmed [31]; antigen-presenting cells in the pancreas present their own antigens, which leads to the activation of helper T cells, followed by macrophages, NK cells, and cytotoxic B and T lymphocytes.
Activated cells begin to infiltrate pancreatic tissue (insulitis) [32, 33] in 2 stages, and β-cell destruction occurs through apoptosis mediated by pro-inflammatory cytokines (IL-1, TNF-α, IFN-γ) and activation of transcription factors (NF-κB, STAT-1) [34, 35].
The role of NK cells in the complex pathogenesis of T1DM is probably related to the ability to kill target cells and interact with antigen presenting cells and T cells [22–24]. It has been confirmed in vitro that NK cells can lyse β cells [15, 36, 37].
One of the hypotheses concerning the involvement of NK cells in the development of diabetes is their activation by IL-12. The whole process begins with the macrophages detecting the environmental factor and the entry of these cells into the pancreatic islets, where they then produce IL-12 and IL-18. IL-12 stimulates NK cells to produce IFN-γ, which activates an autoimmune, diabetogenic T-cell response [38].
Another mechanism of action of NK cells is the modulation of the immune response by the secretion of pro-inflammatory cytokines, including IFN-γ. The shifting of the equilibrium towards the pro-inflammatory response leads to a rapid progression from insulitis to full-blown T1DM. Recent papers have underlined both the destructive role and the protective role of NK cells in the pathogenesis of autoimmune diseases [20, 21, 36, 39–42]. Our observation confirms the probably protective role of circulating NK cells because in children with T1DM lower amounts of this were observed. Gomez-Munoz in his research described the decline of NK cells in different stages of the disease. This may suggest extravasation of these cells into the islets – it persisted throughout the detachment period. The number of NKreg cells increased in the early stages after the onset of T1DM. Finally, changes in CD16 expression at different stages of T1DM were identified, highlighting the reduction in CD16 expression in total NK cells and NKeff cells one year after diagnosis. This may reflect a state of exhaustion after repeated interactions between cells compared to healthy siblings and healthy control children.
To properly perform their function NK cells must maintain a balance between inhibitory and excitatory receptors. Expression of MHC class I molecules that suppresses immune responses is involved in recognizing cells as self. Viral infections, especially enteroviruses, may reduce their expression, which results in disinhibition of NK cell activity or, conversely, cause overexpression of class I MHCs, and then other processes enabling immune reactions are stimulated in NK lymphocytes, e.g. the use of cytotoxicity receptors such as NKp46 [38].
NK cells constitute the main population in the infiltrate. Insulitis leads to β-cell dysfunction and impaired insulin secretion. Studies on an animal model and among patients with T1DM have proven that NK cells are involved in the progression of T1DM and can play a protective role in pancreatic islet cells. This suggests that the NK cell population may become a potential therapeutic target in T1DM [43, 44].
Moreover, Semeraro et al. investigated the role of NK cell responses to viral infection and its impact on the pathogenesis of T1DM. They described the effect of a pro-inflammatory mediator called 12/15-lipoxygenase (12/15-LO) in NOD mice and noticed that its high level reduces the number of stimulating receptors on NK cells. They showed a correlation between the expression of 12/15-LO causing the malfunction of NK lymphocytes and the progression of T1DM. It probably occurs as a result of excessive production of IFN-γ, imbalance between stimulating and inhibitory receptors on the NK surface, and excessive autoimmune response from T lymphocytes [38].
The low amount circulating in peripheral blood NK cells may be connected with the concentration of NK in the pancreas. In an animal model, Alba et al. showed that the presence of NK cells is necessary for the development of T1DM. In animals with an accelerated onset of T1DM, an increased percentage of pancreatic NK cells and an increase in the expression of cytokines related to NK cell function and migration were noted. In addition, the removal of the NK cell population in the tested animals resulted in a complete abolition of the acceleration of the autoimmune process. The above study shows the role of viral infections in acceleration of T1DM and the importance of NK cells in this process [27]. Similar results were obtained by Flodstrom et al., confirming the key role of NK cells in the development of insulitis in an animal model [26]. The results of the study by Poirot et al. indicate that increased NK cell infiltration in insulitis is associated with more aggressive islet changes, and removal of the NK cell population has a protective effect on pancreatic β cells [25].
Different results were obtained by Beilke et al. Although researchers reported an increased percentage of pancreatic infiltrating NK cells with an activated cell surface phenotype, it was noted that depriving animals of the NK cell population had no effect on dendritic cell maturation or T-cell proliferation. Thus, NK cells did not influence the onset of T1DM disclosure [45].
On the other hand, Gazali et al. did not observe any changes in the frequency of NK cells in children with T1DM [46].
Gardner et al. noticed in their study that the pancreatic tissue destruction was much worse in mice injected with a low dose of the virus compared to those receiving a high dose of the virus, which was contrary to assumptions. When high doses of virus were applied, the observed effect correlated with much higher activation of NK cells and lower levels of tissue antigen-specific CD8+ T cells. The NK cells were directly cytotoxic to auto-reactive CD8+ T cells in vitro, taken in high-dose blood samples. The exact mechanism appears to be dependent on the expression of NCR1 receptor (NKp46), while the expression of the NKG2D receptor was elevated similarly at both dosages of the virus, and its expression was elevated only in those NK cells in the case of high doses. A study in NCR1 knockout mice infected with LCMV confirmed the observed mechanism of CD8+ T-cell regulation [31]. In our study elevated levels of autoantibodies against elements of β cells and a low percentage of NK cells in children with T1DM suggested that the NK cell subset is engaged in the destructive phase of T1DM.
Kim et al. described the relationship between diabetes (both type 1 and 2) and decreased cytotoxic activity of NK cells. Using mouse models, they showed that longer disease duration was associated with greater reductions in NK activity. Moreover, they observed decreased expression of granzyme B in natural killer cells in mice with T1DM compared to the control group. High levels of expression were noted in 60.8% and 33.8% of NK cells, respectively. Additionally, on the surface of NK lymphocytes in diseased mice, decreased expression of natural cytotoxicity receptors such as NKp46, NKp44, and NKp30 was noted [47].
Ogasawara et al. assessed the activity of NK cells by examining the presence of NKG2D surface receptors and their relationship with T1DM progression. NK cells from the NOD mouse strain have been reported to have low expression of the NKG2D activating receptor. Therefore, the reduced sensitivity of NK cells is likely to be a consequence of “downregulation” by ligands present in pancreatic islets [31, 48]. Moreover, NKG2D blockade acts preventively by delaying the development of T1DM by impairing the function of autoreactive lymphocytes [49].
Similarly, Dong et al. noted the transient downregulation of NK in patients receiving low doses of IL-2 followed by the recovery phase. In their work, they indicated several studies showing the induction of proliferation of various cells of the immune system (including NK cells) by small doses of IL-2 (0.04–1.5 million IU/m2, 3 million IU/m2 and 0.33–1 milion IU/m2). They also cited a study in which dosing 0.125, 0.250, and 0.5 million IU/m2 of IL-2 for 5 days and then once every 2 weeks for a year did not show a difference in NK cell counts compared to those taking placebo [50–53].
However, Qin et al. in their experiment observed that activated NK cells from diabetic patients had similar levels of NKG2D to NK cells from the control group. According to them, this proves that type 1 diabetic NK cells do not downregulate NKG2D ligands after activation, and this in turn suggests that the impairment of NKG2D function may be due to an intracellular defect in signal transduction near the receptor and not from insufficient receptor expression [54].
The ability to activate NK cells was also assessed by Gur et al. The aim of their study was to assess the NKp46 receptor, one of the main activation receptors. NK cell activation occurs as a result of NKp46 fusion with unknown ligands on pancreatic β cells. The authors showed that the lack of NKp46 receptor, or blocking its activity, inhibits the development of T1DM [28, 55]. Aboelnazar et al. also assessed the expression of NK and NKp46+ cells. Cell growth was observed in the group of T1DM mice, but there was no significant difference between those injected with empty chitosan nanoparticles. However, they observed a significant decrease in NK cells in mice injected with a low dose of IL-2 and in those injected with a low dose of IL-2 loaded into chitosan nanoparticles. Also, no significant difference was noticed between the latter group and the non-diabetic group. In contrast, mice injected with a low dose of IL-2 saw a change in NKp46 expression, and the group that was given chitosan with a low dose of IL-2 experienced a significant increase [56]. The protective effect of NK cells in the development of T1DM was described by Lee et al. In an animal model, they proved that a single injection of Freund's Complete Adjuvant (CFA) has a protective effect on pancreatic β cells. CFA causes a rapid increase in the percentage of NK cells and their secretion of IFN-γ [57]. Similar results were obtained by Fidan et al., who noted a higher concentration of IFN-γ among rats with T1DM [58].
Qin et al. in their research also noted that NK cells treated with CFA could protect NOD SCID mice from developing diabetes. It follows that NOD NK cells chronically exposed to NKG2D ligands are desensitized, and their function is increased, which protects NOD mice against disease [54].
Zhou et al. also proved that the protective effect of NK cells with the NK3-like phenotype on pancreatic islets depends on the shifting of the immune response towards Th2 by NK cells acting in a TGF-β-dependent mechanism [30]. To better understand the role of NK cells in the pathogenesis of T1DM, besides determining the percentage of cells in the peripheral blood, it is necessary to assess the number and activity of cells at the site affected by the autoimmune reaction in the pancreas. Brauner et al. assessed the activity of NK cells from the infiltration of pancreatic β cells. In the first phase of the disease, increased activity and pro-inflammatory activity of NK cells were found, and later, hyporeactivity secondary to “exhaustion” [59].
Research that allowed the identification of NK cells with a specific phenotypic profile in the pancreas of NOD mice was conducted by Brauner et al. (2010). Hypothesizing that NK cells are involved in the initiation of the inflammatory process in the pancreas was allowed because the NK cells were characterized by reduced functionality in relation to NK cells of the spleen. Hyporeactivity might take place after the initial phase, due to exhaustion or regulation [60].
Based on the references, it seems that during the period of prediabetes a reduced number of NK cells is observed, and NK cells are involved in the complex pathogenesis of T1DM, especially in the early elimination of damaged or virus-infected β cells. It is likely that insufficient destruction of pancreatic islet cells damaged by viruses leads to the presentation of autoantigens to cells of the immune system. An overview of the above literature is presented in Table III.
Table III
The role of NK cells in the pathogenesis of T1DM – review of the literature
In our study, the lowest number of NK cells was found in the group of children with T1DM, and the highest was found in the control group, while in the group of siblings of diabetic patients, the number of NK cells was average. The obtained results are consistent with the data from the literature described above and confirm the participation of NK cells in the pathogenesis of T1DM. Moreover, the results of our own studies show that in the group of siblings of diabetic patients, the age of children correlated with the number of NK cells. The younger the child, the lower the percentage of CD16+ CD56+ cells. There may be a relationship between the percentage of NK cells and the increased incidence of T1DM among younger children. The analysis of the ROC curves showed that the percentage of NK cells is an effective marker for differentiating between healthy children and children with T1DM. The lower the percentage of NK cells in the peripheral blood, the greater the risk of T1DM in the studied children.
Our observation of a positive correlation between the reduced percentage of NK cells and the elevated levels of anti-GAD and anti-ZnT8 antibodies in children with T1DM, and the lack of this correlation in children without T1DM, may be connected with decreased protective function of NK cells in the early stages of T1DM [20, 21, 36, 39–42].
Conclusions
The reduced percentage of NK cells in children with T1DM and in their siblings compared to the control group may suggests the role of NK cells in the early pathogenesis of T1DM. Genetic predisposition and dysfunction of NK cells probably underlie the pathogenesis of T1DM.
Determination of the NK cell subpopulation in peripheral blood can be a method of selecting a group of patients at risk of developing T1DM among siblings of diabetic patients and healthy children.